How to achieve sustainable antibiotic-free broiler production
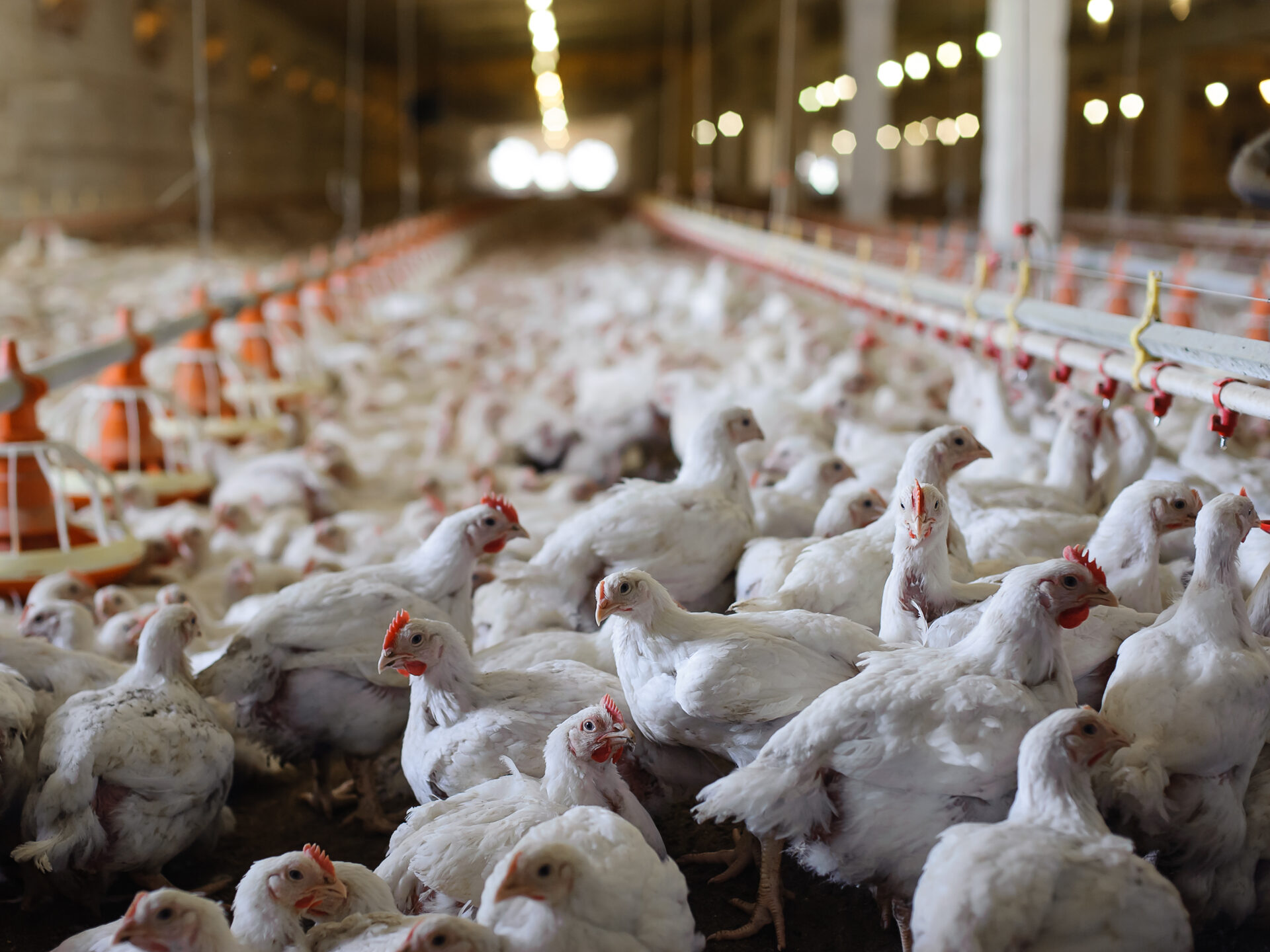
by Predrag Persak, Regional Technical Manager North Europe, EW Nutrition
The main sustainability challenge for broiler production lies in securing enough high-quality, nutritious, safe, and readily available food at a reasonable cost. At times, feed ingredients have to be included that are not nutritionally ideal and might compromise one’s broilers’ health and wellbeing. However, counteracting this threat with prophylactic antibiotics is not acceptable: We must minimize the use of antibiotics to mitigate antimicrobial resistance. The way forward is to go beyond static and linear nutritional value-to-price thinking. A dynamic nutritional strategy focusing on the interdependencies between ingredients, gut, microbiome, and digestion, enables sustainable ABF broiler production.
Sustainable ABF broiler production requires a dynamic, gut health-oriented nutritional strategy
Sustainability vs. ABF production – is there a trade-off?
The United Nations’ 1987 Brundtland report offers a clear definition of sustainability as “development that meets the needs of the present without compromising the ability of future generations to meet their own needs.” “Ability” includes the availability of resources – and in broiler production, which is one of the most efficient livestock productions, resources have always been a top priority. As a constantly evolving industry, broiler production has been quick to adopt sustainability into its management strategies. The use of the resource that is antibiotics, however, poses particular challenges.
Humans and animals depend on antibiotics to fight microbial infections. It is essential to maintain their efficacy so that future generations can lead healthy lives. Antibiotic efficacy is under threat from the development of antimicrobial resistance, which emerges from overuse and misuse in both human and veterinary medicine. Across the globe, broilers are still raised with the assistance of antibiotics. Either for disease therapy, to prevent disease occurrence, and still, in some parts of the world, to enhance performance. Driven by regulatory and consumer demands, broiler production with minimal or no use of antibiotics is rapidly gaining importance.
The challenges of antibiotic-free broiler production
ABF systems encounter numerous challenges since production requirements change drastically. Stock density must be lower; it takes longer to reach the desired weight; and more feed is needed to produce the same amount, with a higher risk of morbidity and mortality (Cervantes, 2015). The latter can result in more birds needing treatment with medically important antimicrobial drugs. All those challenges need to be overcome by adopting suitable strategies related to nutrition, genetics, management, biosecurity, welfare, and food safety.
As animal nutritionists, our focus lies on nutrition, feed, feed materials, additives, feed processing, feeding, and their (positive or negative) influence on the sustainability of ABF broiler production. However, we cannot look at these dimensions of production as a separate process. They are linked in the whole food chain and are affected by changes that happen in other related parts. An obvious example is feed production, which has an enormous impact on the overall sustainability of ABF broiler production:
- Due to raw material shortages, diets are becoming ever more complex, containing more single feed ingredients. For some of them, we need a better understanding of their impact on ABF broiler production (e.g., sunflower, rapeseed, beans, lupins).
- The nutritional composition of raw materials changes due to limitations in fertilizer use, and variability within the same raw material group is expected to increase.
- New food waste-reducing feed materials can enhance feed security but also require nutritional profiling to integrate them into diets.
- Local feed material production in humid and warm environments can introduce various pathogens into the feed/food chain.
- Increases in known and the emergence of new antinutrients and feed components that impair animal health, performance, and feed efficiency.
- Sustainability-driven pesticide reduction raises concerns about mycotoxins contaminating feed ingredients.
- Nutrient reduction to support gut health and, primarily, lower the excretion of nitrogen and phosphorous, negatively affects growth, nutritional standards, and the ability to freely select feed materials to include in broiler diets.
- The value (of which price is also part) of raw materials will be compromised, due to availability and nutritional variability.
Mycotoxin contaminated-feed can damage production animals’ performance, health, and welfare
When striving for a sustainable ABF broiler production approach, the possibility for errors becomes higher, while the error margin becomes smaller. The solution lies in helping the animals to mitigate the impact of stressors by focusing on the interaction of ingredients, gut, microbiome, and digestion. It is a holistic approach centered on gut health. Keeping the intestines BEAUTIful will help you produce in challenging conditions without the use of antimicrobials.
Keep the broiler gut BEAUTIful and resilient to stress
The BEAUTIful formula captures the six areas producers need to target for supporting broiler gut health:
Barrier
If it’s working correctly, the effective gatekeeper knows what gets in and what stays out. When the barrier function is compromised due to stress, pathogens can cause infections, disrupt health, and negatively impact broiler immunity. Necrotic enteritis, femoral head necrosis, and bacterial chondronecrosis with osteomyelitis (BCO) are common diseases that affect today’s broiler production (Wideman, 2015). As the source of nutrients, feed serves as a modulator of various physiological functions in the intestinal tract, including intestinal barrier function.
Enzymatic digestion
The gut is where endogenous and exogenous enzymes perform their hydrolysis functions to break down complex nutrients into the parts that can be used either by the intestinal tissue itself or for the whole animal. One part of hybrid enzymatic digestion is the fermentation by commensal microbes, in which complex materials form end-products of high biological values (such as short-chain fatty acids, SCFA).
Absorption
Maintaining the gut’s resorptive capacity is essential to secure the total intake of digested nutrients. Otherwise, pathogenic bacteria might use the excess nutrients to grow, form toxins, and affect the birds’ health and productivity.
United microbiome
The intestine of a broiler chicken is colonized by more than 800 species of bacteria and other inhabitants, such as viruses and simple organisms that are still unknown. By competitive exclusion and secretion of bacteriocins (volatile fatty acids, organic acids, and natural antimicrobial compounds), commensal bacteria keep the host safe from an overgrowth of dangerous bacteria (e.g., Salmonella, Campylobacter, and Clostridium perfringens). The fine-tuned diversity in the intestinal flora and balance in all interactions between it, the host, and the ingesta are needed for birds to stay healthy and perform well.
Transport
Birds’ digestive tract volumes are smaller than those of mammals with similar body weight. This means that they achieve more efficient nutrient digestion in a shorter retention time, averaging between 5 and 6 hours. Passing the small intestine usually takes around 3 hours, of which 1 hour is spent in the duodenum and jejunum. Transport times are affected by the feeding system and the extent to which material enters the caeca. Reflux of material from the distal to the proximal small intestine is an important feature that helps digestion and maintenance of a healthy gut.
Immunity
The intestinal microbiota is critically important for the development and stimulation of the immune system. The intestine is the key immunological organ, comprised of myeloid and lymphoid cells, and a site for producing many immune cell types needed to initiate and mediate immunity. Together with the microbiome, dendritic cells induce antigen-specific responses and form immunoglobulin A, which works in the intestinal lumen.
Natural gut health solution for sustainable ABF broiler production
In practice, supporting broiler gut health requires a holistic approach that includes natural feed additive solutions. Phytomolecules are compounds that certain plants develop as defenses mechanisms. Phytomolecules-based solutions should feature prominently in sustainable ABF broiler production approaches due to their advantageous properties:
Enhance digestion, manage variability
Sustainability necessitates efficient resource utilization. Digestion support needs to be a priority to use the available feed in its entirety. This is particularly important if antibiotics use needs to be minimized: a maximum of nutrients should be utilized by the animal; otherwise, they feed potentially harmful bacteria, necessitating antibiotic treatments. Enhancing digestibility is the focus when we are dealing with variable feed materials or feed changes that represent stress to the animal. Selected phytomolecules have proven efficient at improving performance due to enhanced digestion (Zhai et al. 2018).
Work on microbiome and pathogens
The antimicrobial activity of certain phytomolecules can prevent the overgrowth of pathogens in the gastrointestinal tract, thereby reducing dysbacteriosis (Liu et al., 2018) and specific diseases such as necrotic enteritis. Studies on broilers show that they also reduce the adhesion of pathogens to the wall of the intestine. Certain phytomolecules even possess antimicrobial characteristics against antibiotic-resistant pathogens.
Keep gut integrity
Phytomolecules help maintain tight junction integrity, thus preventing leaky gut (Li et al., 2009). As a result, the potential flow of bacteria and their toxins from the gut lumen into the bloodstream is mitigated. Their properties thus make phytomolecules a promising alternative to the non-therapeutic use of antibiotics.
Trial results: Phytomolecules enhance broiler gut health
To test the efficacy of phytomolecules, we conducted a large-scale field study in Brazil, under practical conditions. The focus was on growth performance, and no growth-promoting antibiotics were used. Lasting 5 months, the trial involved more than 2 million broilers. The birds were divided into a control and a trial group, with two repetitions per group. Both groups were fed the standard feed of the farm. The trial group additionally received 100g of Activo per MT in its finisher feed for 3 weeks. The study clearly shows that Activo supplementation improves performance parameters (daily weight gain, average total gain, and improved feed efficiency), which resulted in a higher production efficiency factor (PEF):
- Activo groups had a 3 % higher average daily weight gain and reached their slaughtering age earlier
- The final weight of Activo groups was about 2.5 % higher than in the control group
- With a 2 points better feed conversion, the animals of the Activo group achieved a 13.67 points higher PEF
Figure 1: Broiler performance results, Activo vs. non-supplemented control group
Conclusion
Antibiotic-free broiler production is a challenging endeavor: producers need to maintain animal welfare and keep up efficiency while making farming profitable. Over time, these challenges will affect producers even more as sustainability requirements increase across all parts of the broiler production chain. On top of that, coccidiostats, which are essential for efficient broiler production, are increasingly being questioned, which will require concerted research into feed additive solutions.
To make sustainable ABF broiler production the norm, it is unavoidable to adopt suitable strategies related to nutrition, genetics, management, biosecurity, welfare, and food safety. Effective, scientifically and practically proven tools already exist: Thanks to their positive impact on intestinal health, phytomolecules reliably support sustainable broiler production without antibiotics.
References
Cervantes, Hector M. “Antibiotic-Free Poultry Production: Is It Sustainable?” Journal of Applied Poultry Research 24, no. 1 (2015): 91–97. https://doi.org/10.3382/japr/pfv006.
Li, Y., H.Y. Cai, G.H. Liu, X.L. Dong, W.H. Chang, S. Zhang, A.J. Zheng, and G.L. Chen. “Effects of Stress Simulated by Dexamethasone on Jejunal Glucose Transport in Broilers.” Poultry Science 88, no. 2 (2009): 330–37. https://doi.org/10.3382/ps.2008-00257.
Liu, ShuDong, MinHo Song, Won Yun, JiHwan Lee, ChangHee Lee, WooGi Kwak, NamSoo Han, HyeunBum Kim, and JinHo Cho. “Effects of Oral Administration of Different Dosages of Carvacrol Essential Oils on Intestinal Barrier Function in Broilers.” Journal of Animal Physiology and Animal Nutrition 102, no. 5 (2018): 1257–65. https://doi.org/10.1111/jpn.12944.
Wideman, Robert F. “Bacterial Chondronecrosis with Osteomyelitis and Lameness in Broilers: a Review.” Poultry Science 95, no. 2 (2016): 325–44. https://doi.org/10.3382/ps/pev320.
Zhai, Hengxiao, Hong Liu, Shikui Wang, Jinlong Wu, and Anna-Maria Kluenter. “Potential of Essential Oils for Poultry and Pigs.” Animal Nutrition 4, no. 2 (2018): 179–86. https://doi.org/10.1016/j.aninu.2018.01.005.