Price hikes = more cereal byproducts in animal feed. What about mycotoxin risk?
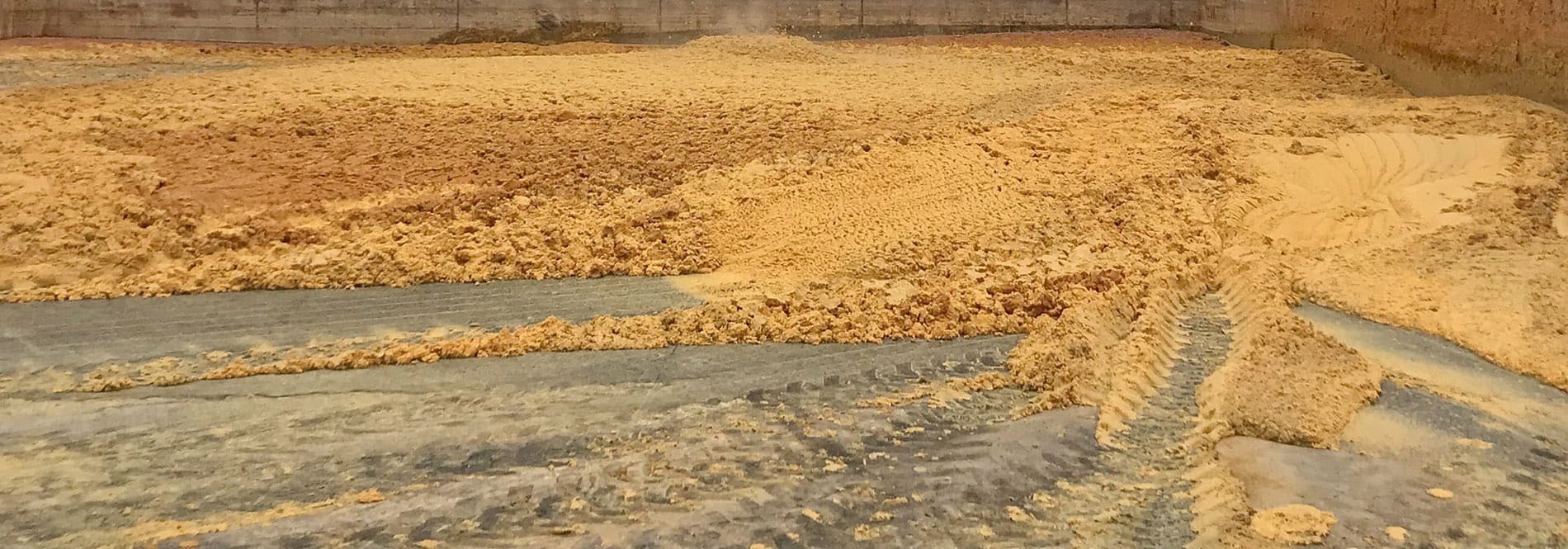
By Marisabel Caballero, Global Technical Manager Poultry, EW Nutrition
Most grains used in feed are susceptible to mycotoxin contamination, causing severe economic losses all along feed value chains. As skyrocketing raw material prices force producers to include a higher proportion of economical cereal byproducts in the feed, the risks of mycotoxin contamination likely increase. In this article, we review why mycotoxins cause the damage they do – and how effective toxin-mitigating solutions prevent this damage.
Mycotoxin contamination of cereal byproducts requires solutions
Cereal byproducts may become more important feed ingredients as grain prices increase. But also from a sustainability point of view and considering population growth, using cereal byproducts in animal feed makes a lot of sense. Dried distiller’s grains with solubles (DDGS) are a good example of how byproducts from food processing industries can become high-quality animal feed.
Figure 1: Byproducts are a crucial protein source (data from FEFAC Feed & Food 2021 report)
Still, research on what happens to mycotoxins during food processing shows that mycotoxins are concentrated into fractions that are commonly used as animal feed (cf. Pinotti et al., 2016 + link to article IH+MC ). To safeguard animal health and performance when feeding lower-quality cereals, it is essential to monitor mycotoxin risks through regular testing and to use toxin-mitigating solutions.
Problematic effects of mycotoxins on the intestinal epithelium
Most mycotoxins are absorbed in the proximal part of the gastrointestinal tract. This absorption can be high, as in the case of aflatoxins (ca. 90%), but also very limited, as in the case of fumonisins (< 1%); moreover, it depends on the species. Importantly, a significant portion of unabsorbed toxins remains within the lumen of the gastrointestinal tract.
Importantly, studies based on realistic mycotoxin challenges (e.g., Burel et al., 2013) show that the mycotoxin levels necessary to trigger damaging processes are lower than the levels reported as safe by EFSA, the Food Safety Agency of the European Union. The ultimate consequences range from diminished nutrient absorption to inflammatory responses and pathogenic disorders in the animal (Figure 2).
Figure 2: Mycotoxins’ impact on the GIT and consequences for monogastric animals
-
Alteration of the intestinal barrier‘s morphology and functionality
Several studies indicate that mycotoxins such as aflatoxin B1, DON, fumonisin B1, ochratoxin A, and T2, can increase the permeability of the intestinal epithelium of poultry and swine (e.g. Pinton & Oswald, 2014). This is mostly a consequence of the inhibition of protein synthesis.
As a result, there is an increase in the passage of antigens into the bloodstream (e.g., bacteria, viruses, and toxins). This increases the animal’s susceptibility to infectious enteric diseases. Moreover, the damage that mycotoxins cause to the intestinal barrier entails that they are also being absorbed at a higher rate.
-
Impaired immune function in the intestine
The intestine is a very active immune site, where several immuno-regulatory mechanisms simultaneously defend the body from harmful agents. Immune cells are affected by mycotoxins through the initiation of apoptosis, the inhibition or stimulation of cytokines, and the induction of oxidative stress.
For poultry production, one of the most severe enteric problems of bacterial origin is necrotic enteritis, which is caused by Clostridium perfringens toxins. Any agent capable of disrupting the gastrointestinal epithelium – e.g. mycotoxins such as DON, T2, and ochratoxin – promotes the development of necrotic enteritis.
-
Alteration of the intestinal microflora
Recent studies on the effect of various mycotoxins on the intestinal microbiota show that DON and other trichothecenes favor the colonization of coliform bacteria in pigs. DON and ochratoxin A also induce a greater invasion of Salmonella and their translocation to the bloodstream and vital organs in birds and pigs – even at non-cytotoxic concentrations.
It is known that fumonisin B1 may induce changes in the balance of sphingolipids at the cellular level, including for gastrointestinal cells. This facilitates the adhesion of pathogenic bacteria, increases in their populations, and prolongs infections, as has been shown for the case of E. coli. The colonization of the intestine of food-producing animals by pathogenic strains of E. coli and Salmonella also poses a risk for human health.
-
Interaction with bacterial toxins
When mycotoxins induce changes in the intestinal microbiota, this can lead to an increase in the endotoxin concentration in the intestinal lumen. Endotoxins promote the release of several cytokines that induce an enhanced immune response, causing inflammation, thus reducing feed consumption and animal performance, damage to vital organs, sepsis, and death of the animals in some cases.
The synergy between mycotoxins and endotoxins can result in an overstimulation of the immune system. The interaction between endotoxins and estrogenic agents such as zearalenone, for example, generates chronic inflammation and autoimmune disorders because immune cells have estrogen receptors, which are stimulated by the mycotoxin.
Increased mycotoxin risks through byproducts? Invest in mitigation solutions
To prevent the detrimental consequences of mycotoxins on animal health and performance, proactive solutions are needed that support the intestinal epithelium’s digestive and immune functionality and help maintain a balanced microbiome in the GIT. As the current market conditions will likely engender a long-term shift towards the inclusion of more cereal byproducts in animal diets, this becomes even more important.
Trial data shows that EW Nutrition’s toxin-mitigating solution SOLIS MAX provides effective protection against feedborne mycotoxins. The synergistic combination of ingredients in SOLIS MAX mycotoxins from damaging the animals’ gastrointestinal tract and entering the blood stream:
In-vitro study shows SOLIS MAX’ strong mitigation effects against wide range of mycotoxins
Animal feed is often contaminated with two or more mycotoxins, making it important for an anti-mycotoxin agent to be effective against a wide range of different mycotoxins. A dose response evaluation of SOLIS MAX was conducted a at an independent laboratory in Spain, for inclusion levels of 0.10%, 0.15%, and 0.20% (equivalent to 1 kg, 1.5 kb, and 2 kg per ton of feed). A phosphate buffer solution at pH 7 was prepared to simulate intestinal conditions in which a portion of the mycotoxins may be released from the binder (desorption).
Each mycotoxin was tested separately by adding a challenge to buffer solutions, incubating for one hour at 41°C, to establish the base line (see table). At the same time a solution with the toxin challenge and SOLIS MAX was prepared, incubated, and analyzed for the residual mycotoxin. All analyses were carried out by high performance liquid chromatography (HPLC) with standard detectors.
Figure 3: SOLIS MAX adsorption capacity against different mycotoxins (%)
The results demonstrate that SOLIS MAX is a very effective solution against the most common mycotoxins found in raw materials and animal feed, showing clear dose-response effects.
Mycotoxin risk management for better animal feed
A healthy gastrointestinal tract is crucial to animals’ overall health: it ensures that nutrients are optimally absorbed, it provides effective protection against pathogens through its immune function, and it is key to maintaining a well-balanced microflora. Even at levels considered safe by the European Union, mycotoxins can compromise different intestinal functions, resulting in lower productivity and susceptibility to disease.
The globalized feed trade, which spreads mycotoxins beyond their geographical origin, climate change and raw material market pressures only escalates the problem. On top of rigorous testing, producers should mitigate unavoidable mycotoxin exposures through the use of solutions such as SOLIS MAX – for stronger animal health, welfare, and productivity.
References
Antonissen, Gunther, An Martel, Frank Pasmans, Richard Ducatelle, Elin Verbrugghe, Virginie Vandenbroucke, Shaoji Li, Freddy Haesebrouck, Filip Van Immerseel, and Siska Croubels. “The Impact of Fusarium Mycotoxins on Human and Animal Host Susceptibility to Infectious Diseases.” Toxins 6, no. 2 (January 28, 2014): 430–52. https://doi.org/10.3390/toxins6020430.
Burel, Christine, Mael Tanguy, Philippe Guerre, Eric Boilletot, Roland Cariolet, Marilyne Queguiner, Gilbert Postollec, et al. “Effect of Low Dose of Fumonisins on Pig Health: Immune Status, Intestinal Microbiota and Sensitivity to Salmonella.” Toxins 5, no. 4 (April 23, 2013): 841–64. https://doi.org/10.3390/toxins5040841.
Burton, Emily J., Dawn V. Scholey, and Peter E. Williams. “Use of Cereal Crops for Food and Fuel – Characterization of a Novel Bioethanol Coproduct for Use in Meat Poultry Diets.” Food and Energy Security 2, no. 3 (September 19, 2013): 197–206. https://doi.org/10.1002/fes3.30.
Ghareeb, Khaled, Wageha A. Awad, Josef Böhm, and Qendrim Zebeli. “Impacts of the Feed Contaminant Deoxynivalenol on the Intestine of Monogastric Animals: Poultry and Swine.” Journal of Applied Toxicology 35, no. 4 (October 28, 2014): 327–37. https://doi.org/10.1002/jat.3083.
Mani, V., T. E. Weber, L. H. Baumgard, and N. K. Gabler. “Growth and Development Symposium: Endotoxin, Inflammation, and Intestinal Function in livestock1,2.” Journal of Animal Science 90, no. 5 (May 1, 2012): 1452–65. https://doi.org/10.2527/jas.2011-4627.
Obremski, K. “The Effect of in Vivo Exposure to Zearalenone on Cytokine Secretion by Th1 and Th2 Lymphocytes in Porcine Peyer’s Patches after in Vitro Stimulation with LPS.” Polish Journal of Veterinary Sciences 17, no. 4 (2014): 625–32. https://doi.org/10.2478/pjvs-2014-0093.
Oswald, I. P., C. Desautels, J. Laffitte, S. Fournout, S. Y. Peres, M. Odin, P. Le Bars, J. Le Bars, and J. M. Fairbrother. “Mycotoxin Fumonisin B1 Increases Intestinal Colonization by Pathogenic Escherichia Coli in Pigs.” Applied and Environmental Microbiology 69, no. 10 (2003): 5870–74. https://doi.org/10.1128/aem.69.10.5870-5874.2003.
Pinotti, Luciano, Matteo Ottoboni, Carlotta Giromini, Vittorio Dell’Orto, and Federica Cheli. “Mycotoxin Contamination in the EU Feed Supply Chain: A Focus on Cereal Byproducts.” Toxins 8, no. 2 (February 15, 2016): 45. https://doi.org/10.3390/toxins8020045.
Pinton, Philippe, and Isabelle Oswald. “Effect of Deoxynivalenol and Other Type B Trichothecenes on the Intestine: A Review.” Toxins 6, no. 5 (May 21, 2014): 1615–43. https://doi.org/10.3390/toxins6051615.