Optimizing the Use of DDGS in Poultry Feeds with Xylanase
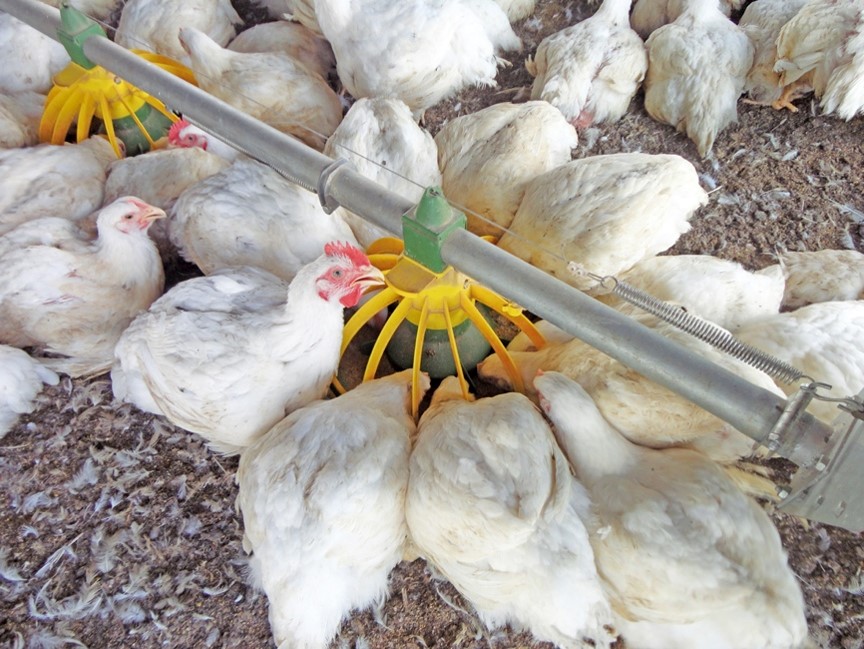
Author: Ajay Bhoyar, Sr. Global Technical Manager, EW Nutrition
As the poultry industry seeks economical and nutritious feed ingredients, distillers’ dried grains with solubles (DDGS), a co-product of grain-based ethanol production, presents a valuable option providing beneficial protein, energy, water-soluble vitamins, xanthophylls, and linoleic acid. However, the inherent variability in DDGS nutrient composition and high fiber content can pose challenges for consistent inclusion in poultry feeds. The strategic use of feed enzymes has become a significant area of focus to overcome these limitations and further enhance the nutritional value of DDGS in poultry diets. This article will explore the optimization of DDGS utilization in poultry feeds by emphasizing the inclusion of xylanase enzyme that can efficiently degrade the insoluble arabinoxylans. By understanding the factors affecting DDGS quality and strategically employing xylanase, poultry producers can potentially achieve higher inclusion rates of this readily available byproduct, aiming to reduce feed costs while maintaining or even improving production performance and overall health.
Price competitiveness of DDGS
The price of DDGS relative to other feed ingredients, primarily corn and soybean meal, is a significant factor in its global utilization. DDGS often partially replaces these traditional energy (corn) and protein (soybean meal) sources in animal feeds, leading to significant diet cost savings for poultry producers. DDGS contains a high amount of a combination of energy, amino acids, and phosphorus. However, it is usually undervalued as its price is mainly determined based on the prevailing prices of corn and soybean meal.
Variability in the nutritional quality of DDGS
The nutrient composition of DDGS varies based on the starting grain, ethanol production methods, and drying processes. Generally, DDGS contains high levels of protein, fiber, and minerals, with varying amounts of fat and starch depending on the type of grain used and how it is processed. DDGS has a reputation for having variable nutrient composition, protein quality, and a high content of mycotoxins (Stein et al., 2006; Pedersen et al., 2007; Anderson et al., 2012). High quantities of DDGS in feed increase dietary fiber, adversely affecting nutrient digestibility.
The variations in production methods lead to significant differences in the following nutritional components of DDGS:
Crude Fat: This is one of the most variable components, ranging from 5 to 9 percent in reduced-oil DDGS and greater than 10 percent in traditional high-oil DDGS.
Energy: The apparent metabolizable energy (AMEn) for poultry varies among DDGS sources. Fiber digestibility and the digestibility of the extracted oil also contribute to this variability. The high temperatures during the drying stage of DDGS production accelerate lipid peroxidation, forming breakdown products from the fats. This peroxidation contributes to the changes and variability observed in the fat component of DDGS and is a factor that can affect nutrient digestibility and overall energy value.
Crude Protein and Amino Acids (especially Lysine): While crude protein content might not always increase inversely with fat reduction, the digestibility of amino acids, especially lysine, can be affected by drying temperatures. Lysine digestibility of DDGS is a primary concern of poultry nutritionists due to the susceptibility of this amino acid to Maillard reactions during the drying process of DDGS, which can reduce both the concentration and digestibility of lysine (Almeida et al. 2013). Prediction equations have been developed to accurately estimate actual AMEn and standardized ileal digestible amino acid content of DDGS sources based on chemical composition.
Phosphorus: The phosphorus content can vary depending on the amount of Condensed Distiller’s Solubles (CDS) added. The bioavailability of phosphorus can also be influenced by processing. The phosphorus content in the corn DDGS may vary from 0.69 to 0.98 % (Olukosi and Adebiyi, 2013).
Fiber: The neutral detergent fiber (NDF) content is another variable component. Differences in processing conditions among ethanol plants can lead to variations in fiber digestibility.
Table 1. Variation in composition of corn DDGS sources (dry matter basis; adapted from (Pederson et al., 2014)
Analyte | Average | Range |
---|---|---|
Moisture % | 8.7 | 6.5 – 12.5 |
Crude protein % | 31.4 | 27.1 – 36.4 |
Crude fiber % | 7.7 | 6.4 – 9.5 |
Ether Extract % | 9.1 | 6.5 – 11.8 |
NDF % | 35.1 | 30.2 – 39.7 |
ADF % | 10.1 | 8.9 – 11.9 |
Nonstarch Polysaccharides (NSP) in DDGS
Non-starch polysaccharides (NSP) are a significant component of DDGS. The NSP profile of DDGS is crucial for understanding its digestibility and energy content. The corn DDGS has a complex fiber structure that may limit its digestibility in swine and poultry. NSPs in corn DDGS represent 25-34% of its composition, primarily insoluble (Pedersen et al. 2014). The complexity of the fiber structure in corn DDGS makes it more challenging to degrade with enzymes than wheat DDGS. Therefore, while including DDGS in the poultry feeds, choosing an exogenous xylanase enzyme that is highly efficient in breaking down both soluble and insoluble arabinoxylans is essential for maximum energy utilization.
Use of xylanase in DDGS diets for poultry
Supplementing exogenous enzymes in swine and poultry diets have numerous potential benefits including: reduction of digesta viscosity to enhance lipid and protein digestion; increase the metabolizable energy content of the diet; increase feed intake, growth rate and feed conversion; decreased size and alter the microbial population of the gastrointestinal tract; reduce water consumption and water content of excreta in poultry; reduce the amount of excreta as well as ammonia, nitrogen and phosphorus content (Khattak et al., 2006). The selection of a specific enzyme must be based on the type and availability of the target substrate in the diet.
The improved energy utilization of DDGS in poultry can be achieved through the enzymatic degradation of fiber (NSP). Nonstarch polysaccharides within DDGS exist in matrices with starch and protein, so NSP degradation via exogenous enzymes can also release other nutrients for subsequent digestion and absorption (Jha et al. 2015).
The cell wall matrix in corn DDGS is more complex. Moreover, the most readily degradable arabinoxylan for the fiber-degrading enzymes is modified during DDGS production (Pedersen et al. 2014). Many studies reported a greater branch density and complexity of corn arabinoxylan than wheat (Bedford, 1995; Saulnier et al.,1995a; Jilek and Bunzel, 2013; Yang et al., 2013). These observations indicate that the fiber-degrading enzymes applied for the degradation of corn DDGS need to be targeted towards highly complex substrates. This calls for selecting xylanase, which effectively breaks down the insoluble arabinoxylans in diets.
Axxess XY: Highly effective xylanase in breaking down soluble and insoluble arabinoxylans
A bacterial GH10 family xylanase, like Axxess XY, is more beneficial in animal production due to their efficient mechanism of action, broader substrate specificity, and better thermostability. Generally, the GH10 xylanases exhibit broader substrate specificity and can efficiently hydrolyze various forms of xylan, including soluble and insoluble substrates. GH10 xylanases exhibit higher catalytic versatility and can catalyze the cleavage of the xylan backbone at the non-reducing side of substituted xylose residues, whereas GH11 enzymes require unsubstituted regions of the xylan backbone (Collins et al., 2005; Chakdar et al., 2016).
Fig.1. Activity of a bacterial GH10 xylanase against soluble and insoluble arabinoxylans
Axxess XY facilitates DDGS use and reduces the cost of broiler production.
Including xylanase enzyme, which is highly effective in breaking down soluble and insoluble arabinoxylans in poultry feeds, can reduce feed costs, allowing higher inclusion of DDGS while maintaining the bird’s commercial performance.
In a recently conducted 42-day trial at a commercial farm, Axxess XY maintained broiler performance with a 100 kcal/kg reduction in metabolizable energy and 8% use of Corn DDGS in a corn-SBM based diet (Figure 2). This significantly reduced feed cost/kg body weight.
Incorporating DDGS into poultry diets presents a sustainable and cost-effective solution, but its full potential is often limited by variability in nutrient composition and high fiber content. Xylanase enzymes, particularly those in the GH10 family like Axxess XY, can overcome these barriers by breaking down complex arabinoxylans and unlocking inaccessible nutrients. With proven benefits in energy utilization, nutrient digestibility, and overall production efficiency, xylanase inclusion emerges as a strategic approach to optimize DDGS usage, ultimately supporting economic and environmental sustainability goals in poultry production.
References
Almeida, F.N.; Htoo, J.K.; Thomson, J.; Stein, H.H. Amino acid digestibility of heat-damaged distillers’ dried grains with soluble fed to pigs. J. Anim. Sci. Biotechnol. 2013, 4, 2–11.
Bedford, M.R., 1995. Mechanism of action and potential environmental benefits from the use of feed enzymes. Anim. Feed Sci. Technol. 53, 145–155.
Chakdar, Hillol, Murugan Kumar, Kuppusamy Pandiyan, Arjun Singh, Karthikeyan Nanjappan, Prem Lal Kashyap, and Alok Kumar Srivastava. “Bacterial Xylanases: Biology to Biotechnology.” 3 Biotech 6, no. 2 (June 30, 2016). https://doi.org/10.1007/s13205-016-0457-z.
Collins, Tony, Charles Gerday, and Georges Feller. “Xylanases, Xylanase Families and Extremophilic Xylanases.” FEMS Microbiology Reviews 29, no. 1 (January 2005): 3–23. https://doi.org/10.1016/j.femsre.2004.06.005.
Jha, R.; Woyengo, T.A.; Li, J.; Bedford, M.R.; Vasanthan, T.; Zijlstra, R.T. Enzymes enhance degradation of the fiber–starch–protein matrix of distillers dried grains with solubles as revealed by a porcine in vitro fermentation model and microscopy. J. Anim. Sci. 2015, 93, 1039–1051.
Jilek, M.L., Bunzel, M., 2013. Dehydrotriferulic and dehydrodiferulic acid profiles of cereal and pseudocereal flours. Cereal Chem. J. 90, 507–514
Jones, C.K., Bergstrom, J.R., Tokach, M.D., DeRouchey, J.M., Goodband, R.D., Nelssen, J.L., Dritz, S.S., 2010. Efficacy of commercial enzymes in diets containing various concentrations and sources of dried distillers’ grains with solubles for nursery pigs. J. Anim. Sci. 88, 2084–2091.
Khattak, F.M., T.N. Pasha, Z. Hayat, and A. Mahmud. 2006. Enzymes in poultry nutrition. J. Anim. Pl. Sci. 16:1-7.
Olukosi, O.A., and A.O. Adebiyi. 2013. Chemical composition and prediction of amino acid content of maize- and wheat-distillers’ Dried Grains with Soluble. Anim. Feed Sci. Technol. 185:182-189.
Pedersen M. B., Dalsgaard S., Bach Knudsen K.E., Yu S., Lærke H.N., Compositional profile and variation of Distillers Dried Grains with Solubles from various origins with focus on non-starch polysaccharides, Animal Feed Science and Technology, Volume 197, 2014, Pages 130–14.
Saulnier, L., Vigouroux, J., Thibault, J.-F., 1995a. Isolation and partial characterization of feruloylated oligosaccharides from maize bran. Carbohydr. Res. 272,241–253.
Yang, J., Maldonado-Gómez, M.X., Hutkins, R.W., Rose, D.J., 2013. Production and in vitro fermentation of soluble, non-digestible, feruloylated oligo- andpolysaccharides from maize and wheat brans. J. Agric. Food Chem.
Yoon, S.Y., Yang, Y.X., Shinde, P.L., Choi, J.Y., Kim, J.S., Kim, Y.W., Yun, K., Jo, J.K., Lee, J.H., Ohh, S.J., Kwon, I.K., Chae, B.J., 2010. Effects of mannanase and distillers’ dried grain with solubles on growth performance, nutrient digestibility, and carcass characteristics of grower-finisher pigs. J. Anim. Sci. 88,181–191.