Organic acids can play a crucial role in zinc oxide replacement
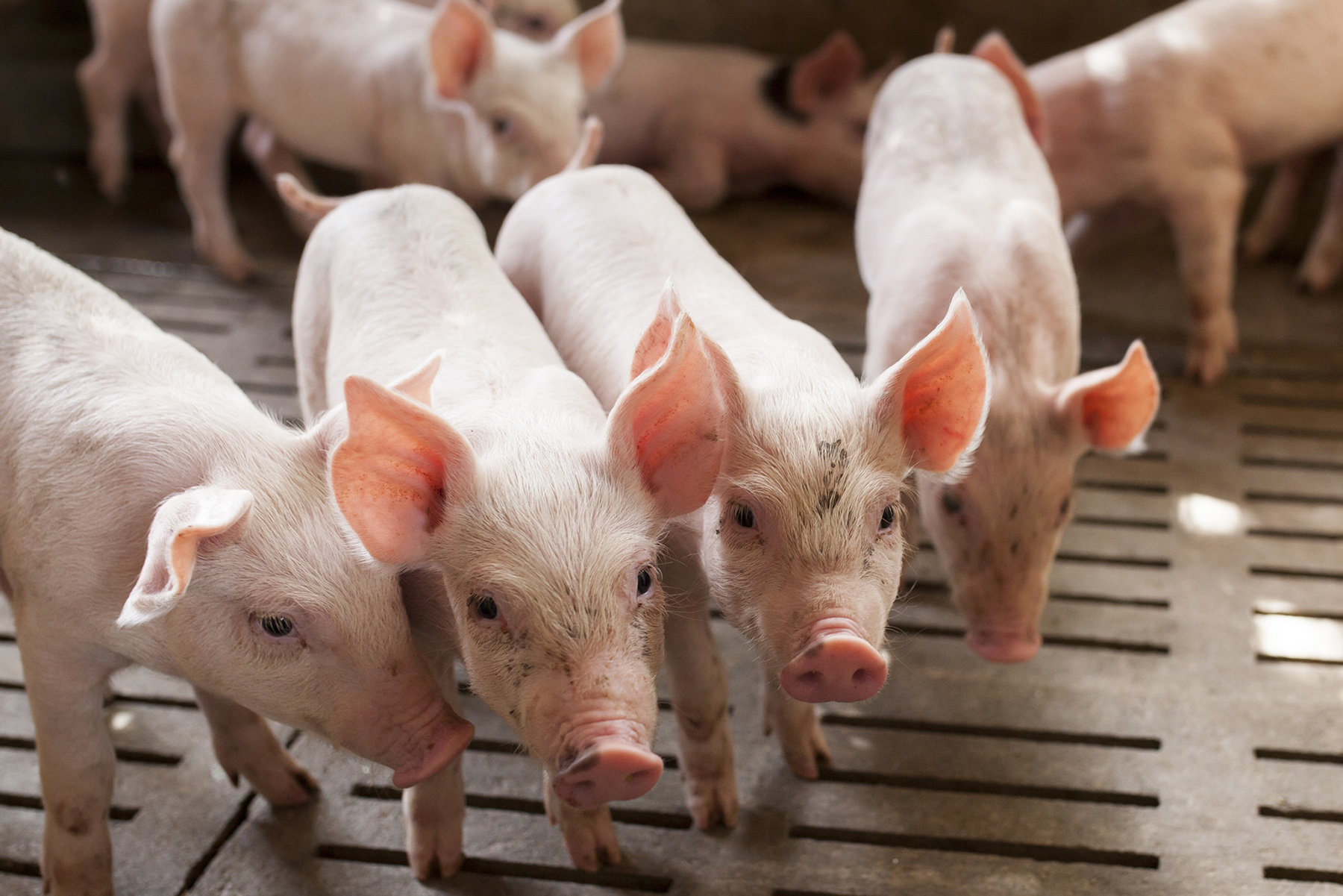
Dr. Inge Heinzl, Editor EW Nutrition &
Juan Antonio Mesonero Escuredo, GTM Swine/GPM Organic Acids EW Nutrition
The use of high levels of Zinc Oxide (ZnO) in the EU before 2022 was one of the most common methods to prevent postweaning diarrhea (PWD) in pig production. Pharmacologically high levels of ZnO (2000-3000 ppm) increase growth and reduce the incidence of enteric bacterial diseases such as post-weaning diarrhea (PWD)( Carlson et al., 1999; Hill et al., 2000; Hill et al., 2001; Poulsen & Larsen, 1995; De Mille et al., 2019).
However, ZnO showed adverse effects, such as the accumulation of heavy metal in the environment, the risk for antimicrobial resistance (AMR), and problems of mineral toxicity and adverse growth effects when feeding it longer than 28 days (Jensen et al., 2018; Cavaco et al., 2011; Vahjen, 2015; Romeo et al., 2014; Burrough et al., 2019). To replace ZnO in pig production, let us first look at its positive effects to know what we must compensate for.
ZnO has a multifactorial mode of action
ZnO shows several beneficial characteristics that positively influence gut health, the immune system, digestion, and, therefore, also overall health and growth performance.
Figure 1. Beneficial effects and ZnO mode of action in postweaning piglets
1. ZnO acts as an antimicrobial
Concerning the antimicrobial effects of ZnO, different possible modes of action are discussed:
- ZnO in high dosages generates reactive oxygen species (ROS) that can damage the bacterial cell walls (Pasquet et al., 2014)
- The death of the bacterial cell due to direct contact of the metallic Zn to the cell (Shearier et al., 2016)
- Intrinsic antimicrobial properties of the ZnO2+ ions after dissociation. The uptake of zinc into cells is regulated by homeostasis. A concentration of the ZnO2+ ions higher than the optimal level of 10-7 to 10-5 M (depending on the microbial strain) allows the invasion of Zn2+ ions into the cell, and the zinc starts to be cytotoxic (Sugarman, 1983; Borovanský et al., 1989).
ZnO shows activity against, e.g., Staphylococcus aureus, Pseudomonas aeruginosa, E. coli, Streptococcus pyogenes, and other enterobacteria (Ann et al., 2014; Vahjen et al., 2016). However, Roselli et al. (2003) did not see a viability-decreasing effect of ZnO on ETEC.
2. ZnO modulates the immune system
Besides fighting pathogenic organisms as described in the previous chapter and supporting the immune system, ZnO is an essential trace element and has a vital role in the immune system. ZnO improves the innate immune response, increasing phagocytosis and oxidative bursts from macrophages and neutrophils. It also ameliorates the adaptative immune response by increasing the number of T lymphocytes (T cells) in general and regulatory T lymphocytes (T-regs) in particular. These cells control the immune response and inflammation (Kloubert et al., 2018). Macrophage capacity for phagocytosis (Ercan and Bor, 1991) and to kill parasites (Wirth et al., 1989), and also the killing activity of natural killer cells depends on Zn (Rolles et al., 2018). By reducing bacterial adhesion and blocking bacterial invasion, ZnO disburdens the immune system (Roselli et al., 2003).
ZnO reduces the expression of several proinflammatory cytokines induced by ETEC (Roselli et al., 2003). Several studies have also shown a modulation effect on intestinal inflammation, decreasing levels of IFN-γ, TNF-α, IL-1ß and IL-6, all pro-inflammatory, in piglets supplemented with ZnO (Zhu et al., 2017; Grilli et al., 2015).
3. ZnO improves digestion and promotes growth
Besides protecting young piglets against diarrhea, the goal is to make them grow optimally. For this target, an efficient digestion and a high absorption of nutrients is essential. Stimulating diverse pancreatic enzymes such as amylase, carboxypeptidase A, trypsin, chymotrypsin, and lipase increases digestibility (Hedemann et al., 2006; Pieper et al., 2015). However, Pieper et al. (2015) also showed that a long-term supply of very high dietary zinc triggers oxidative stress in the pancreas of piglets.
By stimulating the secretion of ghrelin at the stomach level and thereby promoting the release of insulin-like growth factor (IGF-1) and cholecystokinin (CCK), ZnO enhances muscle protein synthesis, cell proliferation, and feed intake (Yin et al., 2009; MacDonald et al., 2000)).
The result of improved digestion is increased body weight and average daily gain, which can be seen, e.g., in a study by Zhu et al. (2017).
4. ZnO protects the intestinal morphology
ZnO prevents the decrease of the trans-endothelial electrical resistance (TEER), usually occurring in the case of inflammation, by downregulating TNF-α and IFN-γ. TNF-α, as well as IFN-γ, increase the permeability of the epithelial tight junctions and, therefore, the intestinal barrier (Al-Sadi et al., 2009).
The enterotrophic and anti-apoptotic effect of ZnO is reflected by a higher number of proliferating and PCNA-positive cells and an increased mucosa surface in the ileum (higher villi, higher villi/crypt ratio)(Grilli et al., 2015). Zhu et al. (2017) also saw an increase in villus height in the duodenum and ileum and a decrease in crypt depth in the duodenum due to the application of 3000 mg of ZnO/kg. Additionally, they could notice a significant (P<0.05) upregulation of the mRNA expression of the zonula occludens-1 and occluding in the mucosa of the jejunum of weaned piglets.
In a trial conducted by Roselli et al. (2003), the supplementation of 0.2 mmol/L ZnO prevented the disruption of the membrane integrity when human Caco-2 enterocytes were challenged with ETEC.
5. ZnO acts antioxidant
The antioxidant effect of ZnO was shown in a study conducted by Zhu et al., 2017. They could demonstrate that the concentration of malondialdehyde (MDA), a marker for lipid peroxidation, decreased on day 14 or 28, and the total concentration of superoxide dismutase (SOD), comprising enzymes that transform harmful superoxide anions into hydrogen peroxide, increased on day 14 (P<0.05). Additionally, Zn is an essential ion for the catalytic action of these enzymes.
Which positive effects of ZnO can be covered by organic acids (OAs)?
1. OAs act antimicrobial
OAs, on the one hand, lower the pH in the gastrointestinal tract. Some pathogenic bacteria are susceptible to low pH. At a pH<5, the proliferation of, e.g., Salmonella, E. coli, and Clostridium is minimized. The good thing is that some beneficial bacteria, such as lactobacilli or bifidobacteria, survive as they are acid-tolerant. The lactobacilli, on their side, can produce hydrogen peroxide, which inhibits, e.g., Staphylococcus aureus or Pseudomonas spp. (Juven and Pierson, 1996).
Besides this more indirect mode of action, a more direct one is also possible: Owing to their lipophilic character, the undissociated form of OAs can pass the bacterial membrane (Partanen and Mroz, 1999). The lower the external pH, the more undissociated acid is available for invading the microbial cells. Inside the cell, the pH is higher than outside, and the OA dissociates. The release of hydrogen ions leads to a decrease in the internal pH of the cell and to a depressed cell metabolism. To get back to “normal conditions”, the cell expels protons. However, this is an energy-consuming process; longer exposure to OAs leads to cell death. The anion remaining in the cell, when removing the protons, disturbs the cell’s metabolic processes and participates in killing the bacterium.
These theoretical effects could be shown in a practical trial by Ahmed et al. (2014). He fed citric acid (0.5 %) and a blend of acidifiers composed of formic, propionic, lactic, and phosphoric acid + SiO2 (0.4 %) and saw a reduction in fecal counts of Salmonella and E. coli for both groups.
2. OAs modulate the immune system
The immune system is essential in the pig’s life, especially around weaning. Organic acids have been shown to support or stimulate the immune system. Citric acid (0.5%), as well as the blend of acidifiers mentioned before (Ahmed et al., 2014), significantly increased the level of serum IgG. IgG is part of the humoral immune system. They mark foreign substances to be eliminated by other defense systems.
Ren et al. (2019) could demonstrate a decrease in plasma tumor necrosis factor-α that regulates the activity of diverse immune cells. He also found lower interferon-γ and interleukin (Il)-1ß values in the OA group than in the control group after the challenge with ETEC. This trial shows that inflammatory response can be mitigated through the addition of organic acids.
3. OAs improve digestion and promote growth
In piglets, the acidity in the stomach is responsible for the activation and stimulation of certain enzymes. Additionally, it keeps the feed in the stomach for a longer time. Both effects lead to better digestion of the feed.
In the stomach, the conversion of pepsinogen to pepsin, which is responsible for protein digestion, is catalyzed under acid conditions (Sanny et al., 1975)group. Pepsin works optimally at two pH levels: pH 2 and pH 3.5 (Taylor, 1959). With increasing pH, the activity decreases; at pH 6, it stops. Therefore, a high pH can lead to poor digestion and undigested protein arriving in the intestine.
These final products of pepsin protein digestion are needed in the lower parts of the GIT to stimulate the secretion of pancreatic proteolytic enzymes. If they do not arrive, the enzymes are not activated, and the inadequate protein digestion continues. Additionally, gastric acid is the primary stimulant for bicarbonate secretion in the pancreas, neutralizing gastric acid and providing an optimal pH environment for the digestive enzymes working in the duodenum.
As already mentioned, the pH in the stomach influences the transport of digesta. The amount of digesta being transferred from the stomach to the small intestine is related to the acidity of the chyme leaving the stomach and arriving in the small intestine. Emptying of the stomach can only take place when the duodenal chyme can be neutralized by pancreatic or other secretions (Pohl et al., 2008); so, acid-sensitive receptors provide feedback regulation and a higher pH in the stomach leads to a faster transport of the digesta and a worse feed digestion.
4. OAs protect the intestinal morphology
Maintaining an intact gut mucosa with a high surface area is crucial for optimal nutrient absorption. Research suggests organic acids play a significant role in improving mucosal health:
Butyric acid promotes epithelial cell proliferation, as demonstrated in an in vitro pig hindgut mucosa study (Sakata et al., 1995). Fumaric acid, serving as an energy source, may locally enhance small intestinal mucosal growth, aiding in post-weaning epithelial cells’ recovery and increasing absorptive surface and digestive capacity (Blank et al., 1999). Sodium butyrate supplementation at low doses influences gastric morphology and function, thickening the stomach mucosa and enhancing mucosal maturation and differentiation (Mazzoni et al., 2008).
Studies show that organic acids affect gut morphology, with a mixture of short-chain and mid-chain fatty acids leading to longer villi (Ferrara et al., 2016) and Na-butyrate supplementation increasing crypt depth and villi length in the distal jejunum and ileum (Kotunia et al., 2004). However, the villi length and mucosa thickness in the duodenum were reduced. Dietary sodium butyrate has been linked to increased microvilli length and cecal crypt depth in pigs (Gálfi and Bokori, 1990).
5. OAs show antioxidant activity
The last characteristic, the antioxidant effect, cannot be provided at the same level as with ZnO; however, Zhang et al. (2019) attest to OAs a certain antioxidant activity. Oxalic, citric, acetic, malic, and succinic acids, which were extracted from Camellia oleifera, also showed good antioxidant activity in a trial conducted by Zhang et al. (2020).
Organic acids are an excellent tool to compensate for the ban on ZnO
The article shows that organic acids have similar positive effects as zinc oxide. They act antimicrobial, modulate the immune system, maintain the gut morphology, fight pathogenic microbes, and also act – slightly – antioxidant. Additionally, they have a significant advantage: they are not harmful to the environment. Organic acids used in the proper pH range and combination are good tools for replacing zinc oxide.
References on request