Dietary interventions for resilient poultry gut health in the AMR era
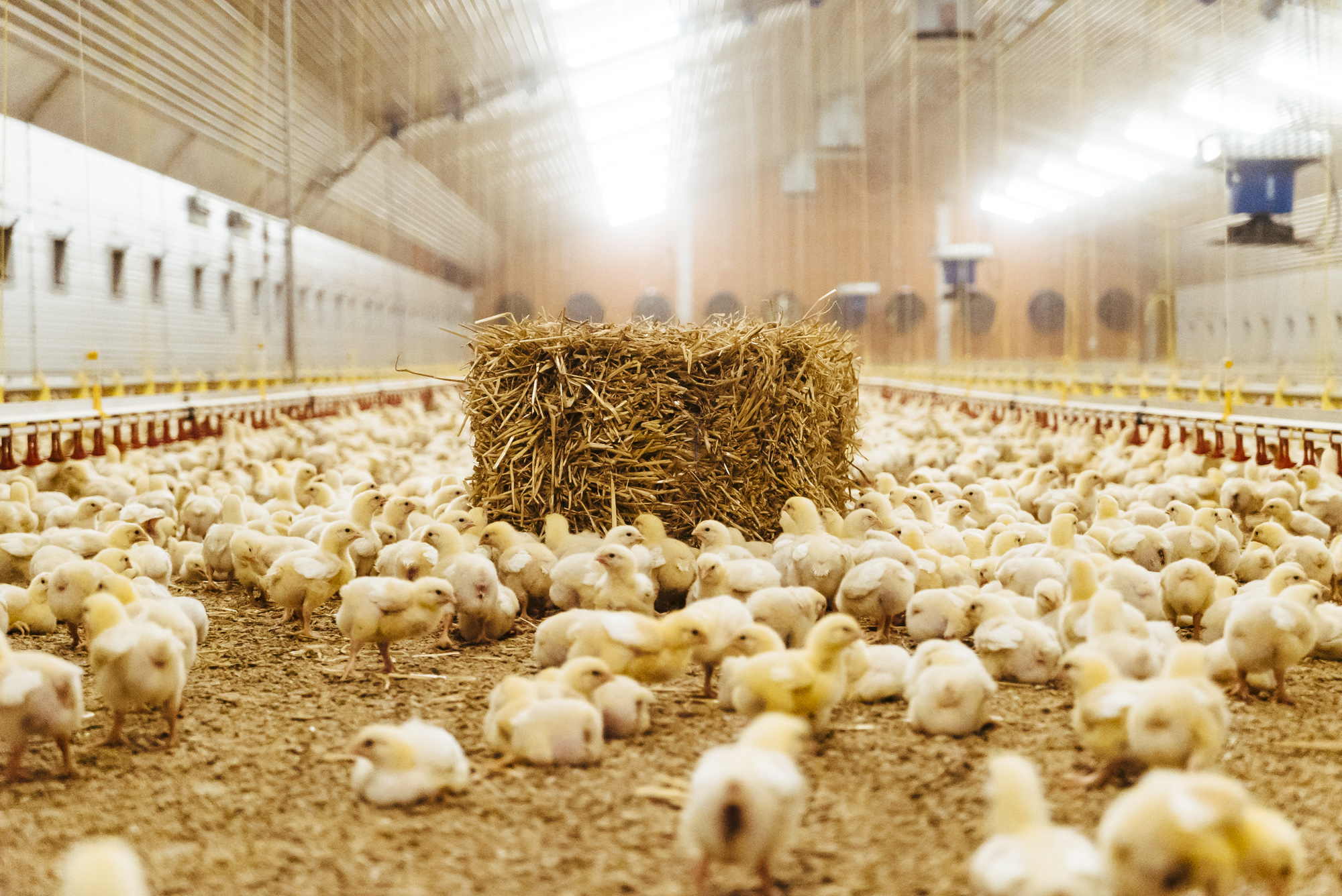
by Ajay Bhoyar, Global Technical Manager, EW Nutrition
Gut health is critical for profitable poultry production, as the gastrointestinal tract (GIT) plays a dual role in nutrient digestion and absorption while serving as a crucial defense against pathogens. A healthy gut enables efficient feed conversion, robust immune function, and resilience against diseases, reducing reliance on preventive and therapeutic antibiotics. Optimum gut health has become increasingly important in poultry production to combat antimicrobial resistance (AMR), a pressing global challenge threatening animal agriculture and public health.
AMR arises when bacteria develop antibiotic resistance, often due to overuse or misuse in human and animal settings. Predictive models suggest that by 2050, AMR could result in 10 million annual deaths and a 2.0%–3.5% reduction in global gross domestic production, amounting to economic losses between 60 and 100 trillion USD. In poultry, AMR compromises flock health, leading to higher mortality, reduced growth performance, and elevated treatment costs, directly impacting profitability. Additionally, resistant pathogens increase the risk of zoonotic disease transfer, posing serious food safety concerns.
Stricter regulations and rising consumer demand for antibiotic-free poultry products drive a shift toward sustainable, antibiotic-free production systems. However, A lack of understanding about strategies to replace AMU and their effectiveness under field conditions hampers change in farming practices (Afonso et al., 2024). Addressing AMR requires a holistic approach, encompassing enhanced biosecurity, innovative health-promoting strategies, and sustainable management practices. This paper explores practical dietary interventions to support poultry gut health while reducing dependency on antimicrobials, offering solutions for the long-term sustainability of poultry production.
Gut Mediated Immunity in Chickens
The gut is a critical component of the immune system, as it is the first line of defense against pathogens that enter the body through the digestive system. Chickens have a specialized immune system in the gut, known as gut-associated lymphoid tissue (GALT), which helps to identify and respond to potential pathogens. The GALT includes Peyer’s patches, clusters of immune cells in the gut wall, and the gut-associated lymphocytes (GALs) found throughout the gut. These immune cells recognize and respond to pathogens that enter the gut.
The gut-mediated immune response in chickens involves several mechanisms, including activating immune cells, producing antibodies, and releasing inflammatory mediators. GALT and GALs play a crucial role in this response by identifying and responding to pathogens and activating other immune cells to help fight off the infection.
The gut microbiome is a diverse community of microorganisms that live in the gut. These microorganisms can significantly impact the immune response. Certain beneficial bacteria, for example, can help stimulate the immune response and protect the gut from pathogens.
Overall, the gut microbiome, GALT, and GALs work together to create an environment hostile to pathogens while supporting the growth and health of beneficial microorganisms.
Key Factors Affecting Poultry Gut Health
The key factors affecting broiler gut health can be summarized as follows:
- Early gut development: Gut-associated immunity responds to early feeding and dietary nutrients and is critical for protecting against exogenous organisms during the first week of life post-hatch.
- Feed and Water Quality: The form, type, and quality of feed provided to broilers can significantly impact their gut health. Consistently available cool and hygienic drinking water is crucial for optimum production performance.
- Stressors: Stressful conditions, such as high environmental temperatures or poor ventilation, can lead to an imbalance in the gut microbiome and an increased risk of disease.
- Infections and medications: Exposure to pathogens or other harmful bacteria can disrupt the gut microbiome and lead to gut health issues. A robust immune system is important for maintaining gut health, as it helps to prevent the overgrowth of harmful bacteria and promote the growth of beneficial bacteria.
- Biosecurity: Keeping the poultry environment clean and free of pathogens is crucial for maintaining gut health, as bacteria and other pathogens can quickly spread and disrupt the gut microbiome.
- Management practices: Best practices, including proper litter management, can help maintain gut health and prevent gut-related issues.
Dietary Interventions for Optimum Gut Health
Gut health means the absence of gastrointestinal disease, the effective digestion and absorption of feed, and a normal and well-established microbiota (Bischoff, 2011). Various dietary measures can be taken to support the healthy functioning of the GIT and host defense. Water and feed safety and quality, feeding management, the form the feed is provided in (e.g., pellets), the composition of the diet, and the use of various feed additives are all tools that can be used to support health (Smits et al., 2021).
Various gut health-supporting feed additives, including organic acids, probiotics, prebiotics, phytochemicals/essential oils, etc., in combination or alone, have been explored as an alternative to antimicrobials in animal production. There were differences in the impacts of the strategies between and within species; this highlights the absence of a ‘one-size-fits-all’ solution. Nevertheless, some options seem more promising than others, as their impacts were consistently equivalent or positive when compared with animal performance using antimicrobials (Afonso et al., 2024). Including insoluble fibers, toxin binders, exogenous enzymes, and antioxidants in the feed formulations also play a crucial role in gut health optimization, which goes beyond their primary functions to combat AMR challenges.
Fig. 1: Multifactorial approach to gut health management in reduced antimicrobial use
Organic Acids
The digestive process extensively includes microbial fermentation, and as a result, organic acids are commonly produced by beneficial bacteria in the crop, intestines, and ceca (Huyghebaert et al., 2010). Organic acids’ inclusion in the poultry diet can improve growth performance due to improved gut health, increased endogenous digestive enzyme secretion and activity, and nutrient digestibility. Butyrate is highly bioactive in GIT. It increases the proliferation of enterocytes, promotes mucus secretion, and may have anti-inflammatory properties (Bedford and Gong, 2018; Canani et al., 2011; Hamer et al., 2008). These effects suggest that it supports mucosal barrier function. Butyrate is becoming a commonly used ingredient in diets to promote GIT health.
Including organic acids in the feed can decontaminate feed and potentially reduce enteric pathogens in poultry. Alternately, the formaldehyde treatment of feed is highly effective at a relatively low cost (Jones, 2011; Wales, Allen, and Davies, 2010).
Organic acids like formic and citric acid are also used in poultry drinking water to lower the microbial count by lowering the water’s pH and preventing/removing biofilms in the water lines. By ensuring feed and water hygiene, producers can minimize pathogen exposure, enhance bird health, and significantly reduce their reliance on antibiotics.
Probiotics, Postbiotics, Prebiotics and Synbiotics
Probiotics and prebiotics have drawn considerable attention to optimizing gut health in animal feeds. Probiotic supplementation could have the following effects: (1) modification of the intestinal microbiota, (2) stimulation of the immune system, (3) reduction in inflammatory reactions, (4) prevention of pathogen colonization, (5) enhancement of growth performance, (6) alteration of the ileal digestibility and total tract apparent digestibility coefficient, and (7) decrease in ammonia and urea excretion (Jha et al., 2020). Certain Lactobacilli or Enterococci species may be used with newly hatched or newborn animals; single or multi-strain starter cultures can be used to steer the initial microbiota in a desired direction (Liao and Nyachoti, 2017). Apart from using probiotics in feed and drinking water, probiotic preparations can be sprayed on day-old chicks in the hatchery or immediately after placement in the brooding house. This way, the probiotic strains/beneficial bacteria gain access to the gut at the earliest possible time (early seeding). Postbiotics are bioactive compounds produced by probiotics during fermentation, such as short-chain fatty acids, peptides, and bacterial cell wall components. Unlike live probiotics, postbiotics are stable, safer, and provide consistent health benefits.
Prebiotics like mannan-oligosaccharides (MOS), inulin, and its hydrolysate (fructo-oligosaccharides: FOS) play an important role in modulating intestinal microflora and potential immune response. Prebiotics reduce pathogen colonization in poultry and promote selective stimulation of beneficial bacterial species. Synbiotics are a combination of probiotics and prebiotics. This synergistic approach offers dual benefits by promoting the growth of beneficial bacteria and directly combating pathogens.
Dietary Fibers (DF)
The water-insoluble fibers are regarded as functional nutrients because of their ability to escape digestion and modulate nutrient digestion. A moderate level of insoluble fiber in poultry diets may increase chyme retention time in the upper part of the GIT, stimulating gizzard development and endogenous enzyme production, improving the digestibility of starch, lipids, and other dietary components (Mateos et al., 2012). The insoluble DF, when used in amounts between 3–5% in the diet, could have beneficial effects on intestinal development and nutrient digestibility.
Dietary fibers influence the development of the gizzard in poultry birds. A well-developed gizzard is a must for good gut health. Jiménez-Moreno & Mateos (2012) noted that coarse fiber particles are selectively retained in the gizzard, ensuring a complete grinding and a well-regulated feed flow. Secretion of digestive juices regulates GIT motility and feed intake. Including insoluble fibers in adequate amounts improves the gizzard function and stimulates HCl production in the proventriculus, thus helping control gut pathogens.
Toxin Risk Management
Mycotoxins may have a detrimental impact on the mucosal barrier function in animals (Akbari et al., 2017; Antonissen et al., 2015; Basso, Gomes and Bracarense, 2013; Pierron, Alassane-Kpembi and Oswald, 2016). Mycotoxins like Aflatoxin B1, Ochratoxin A, and deoxynivalenol (DON) not only suppress immune responses but also induce inflammation and even increase susceptibility to pathogens (Yuhang et al., 2023). To avoid intestinal health problems, poultry producers need to emphasize avoiding levels of mycotoxins in feedstuffs and rancid fats that exceed recommended limits (Murugesan et al., 2015; Grenier and Applegate, 2013).
Bacterial lipopolysaccharides (LPS), also known as endotoxins, are the main components of the outer membrane of all Gram-negative bacteria and are essential for their survival. In stress situations, the intestinal barrier function is impaired, allowing the passage of endotoxins into the bloodstream. When the immune system detects LPS, inflammation sets in and results in adverse changes in gut epithelial structure and functionality. Dietary Intervention to bind these endotoxins in the GIT can help mitigate the negative impact of LPS on animals. Given this, toxin risk management with an appropriate binding agent able to control both mycotoxins and endotoxins appears to be a promising strategy for reducing their adverse effects. Further, adding antioxidants and mycotoxin binders to feed can reduce the effects of mycotoxins and peroxides and is more necessary in ABF programs (Yegani and Korver, 2008).
Essential oils/Phytomolecules
Essential oils (EOs) are important aromatic components of herbs and spices and are used as natural alternatives for replacing antibiotic growth promoters (AGPs) in poultry feed. The beneficial effects of EOs include appetite stimulation, improvement of enzyme secretion related to food digestion, and immune response activation (Krishan and Narang, 2014)
Essential oils (EOs), raw extracts from plants (flowers, leaves, roots, fruit, etc.), are an unpurified mix of different phytomolecules. The raw extract from Oregano is a mix of various phytomolecules (Terpenoids) like carvacrol, thymol and p-cymene. Whereas the phytomolecules are active ingredients of essential oils or other plant materials. Phytomolecule is clearly defined as one active compound.
These botanicals have received increased attention as possible growth performance enhancers for animals in the last decade via their beneficial influence on lipid metabolism, and antimicrobial and antioxidant properties (Botsoglou et al., 2002), ability to stimulate digestion (Hernandez et al., 2004), immune enhancing activity, and anti-inflammatory potential (Acamovic and Brooker, 2005). Many studies have been reported on supplementing poultry diets with some essential oils that enhanced weight gain, improved carcass quality, and reduced mortality rates (Williams and Losa, 2001). The use of some specific EO blends has been shown to have efficacy towards reducing the colonization and proliferation of Clostridium perfringens and controlling coccidia infection and, consequently, may help to reduce necrotic enteritis (Guo et al., 2004; Mitsch et al., 2004; Oviedo-Rondón et al., 2005, 2006a, 2010).
Antimicrobial properties of phytomolecules hinder the growth of potential pathogens. Thymol, eugenol, and carvacrol are structurally similar and have been proven to exert synergistic or additive antimicrobial effects when combined at lower concentrations (Bassolé and Juliani, 2012). In in-vivo studies, essential oils used either individually or in combination have shown clear growth inhibition of Clostridium perfringens and E. coli in the hindgut and ameliorated intestinal lesions and weight loss than the challenged control birds (Jamroz et al., 2006; Jerzsele et al., 2012; Mitsch et al., 2004). One well-known mechanism of antibacterial activity is linked to their hydrophobicity, which disrupts the permeability of cell membranes and cell homeostasis with the consequence of loss of cellular components, influx of other substances, or even cell death (Brenes and Roura, 2010; Solórzano-Santos and Miranda-Novales, 2012; Windisch et al., 2008; O’Bryan et al., 2015).
Apart from use in feed, the liquid phytomolecules preparations for drinking water use can prove to be beneficial in preventing and controlling losses during challenging periods of bird’s life (feed change, handling, environmental stress, etc.). Liquid preparations can potentially reduce morbidity and mortality in poultry houses and thus the use of therapeutic antibiotics. Barrios et al. (2021) suggested that commercial blends of phytomolecule preparations may ameliorate the impact of Necrotic Enteritis on broilers. Further, they hypothesized that the effects of liquid preparation via drinking water were particularly important in improving overall mortality.
In modern, intensive poultry production, the imminent threat of resistant Eimeria looms large, posing a significant challenge to the sustainability of broiler operations. Eimeria spp., capable of developing resistance to traditional anticoccidial drugs, has become a pressing global issue for poultry operators. The resistance of Eimeria to traditional drugs, coupled with concerns over drug residue, has necessitated a shift towards natural, safe, and effective alternatives. It was found that if a drug to which the parasite has developed resistance is withdrawn from use for some time or combined with another effective drug, the sensitivity to that drug may return (Chapman, 1997).
Several phytogenic compounds, including saponins, tannins, essential oils, flavonoids, alkaloids, and lectins, have been the subject of rigorous study for their anticoccidial properties. Among these, saponins and tannins in specific plants have emerged as powerful tools in the fight against these resilient protozoa. Botanicals and natural identical compounds are well renowned for their antimicrobial and antiparasitic activity so that they can represent a valuable tool against Eimeria (Cobaxin-Cardenas, 2016). The mechanisms of action of these molecules include degradation of the cell wall, cytoplasm damage, ion loss with reduction of proton motive force, and induction of oxidative stress, which leads to inhibition of invasion and impairment of Eimeria spp. development (Abbas et al., 2012; Nazzaro et al., 2013). Natural anticoccidial products may provide a novel approach to controlling coccidiosis while meeting the urgent need for control due to the increasing emergence of drug-resistant parasite strains in commercial poultry production (Allen and Fetterer, 2002).
Role of Feed Enzymes Beyond Feed Cost Reduction
Feed enzymes have traditionally been associated with improving feed efficiency and reducing feed costs by enhancing nutrient digestibility. However, their role can extend well beyond economic benefits, profoundly impacting gut health and supporting reduced antimicrobial use in poultry production. Exogenous enzymes reduce microbial proliferation by reducing the undigestible components of feed, the viscosity of digesta, and the irritation to the gut mucosa that causes inflammation. Enzymes also generate metabolites that promote microbial diversity which help to maintain gut ecosystems that are more stable and more likely to inhibit pathogen proliferation (Bedford, 1995; Kiarie et al., 2013).
High dietary levels of non-starch polysaccharides (NSPs) can increase the viscosity of digesta. This leads to an increase in the retention time of the digesta, slows down the nutrient digestion and absorption rate, and may lead to an undesired increase in bacterial activity in the small intestine (Langhout et al., 2000; Smits et al., 1997). Further the mucosal barrier function may also be adversely affected. To solve this problem, exogenous enzymes, such as arabinoxylanase and/ or ß-glucanase, are included in feed to degrade viscous fibre structures (Bedford, 2000). The use of xylanase and ß-glucanase may also cause oligosaccharides and sugars to be released, of which certain, for example, arabinoxylan oligosaccharides, may have prebiotic properties (De Maesschalck et al., 2015; Niewold et al., 2012).
New generation xylanases coming from family GH-10 are known to effectively breakdown both soluble and insoluble arabinoxylans into a good mixture of smaller fractions of arabino-xylo-oligosaccharides (AXOS) and xylo-oligosaccharides (XOS), which exert a prebiotic effect in the GIT. Awati et.al. (2023) observed that a novel GH10 xylanase contributed to positive microbial shift and mitigated the anti-nutritional gut-damaging effects of higher fiber content in the feed. With a substantial understanding of the mode of action and technological development in enzyme technology, nutritionists can reliably consider new-generation xylanases for gut health optimization in their antibiotic reduction strategy.
Conclusions
The challenge of mitigating antimicrobial resistance (AMR) in poultry production necessitates a multidimensional approach, with gut health at its core. Dietary interventions, such as organic acids, probiotics, prebiotics, phytomolecules, toxin binders, and feed enzymes, promote gut resilience, enhance immune responses, and reduce reliance on antimicrobials. These strategies not only support the health and productivity of poultry but also address critical global issues of AMR and food safety.
While no single solution fits all circumstances, integrating these dietary tools with robust biosecurity measures, sound management practices, and continued research on species-specific and field-applicable strategies can pave the way for sustainable, antibiotic-free poultry production. The transition to such systems aligns with regulatory requirements and consumer expectations while contributing to global efforts against AMR.
Ultimately, embracing holistic and innovative dietary strategies ensures a resilient gastrointestinal environment, safeguarding poultry health and productivity while protecting public health and environmental sustainability for future generations.
References: The references can be made available upon request to the author.