From sub-acute ruminal acidosis to endotoxins: Prevention for lactating cows
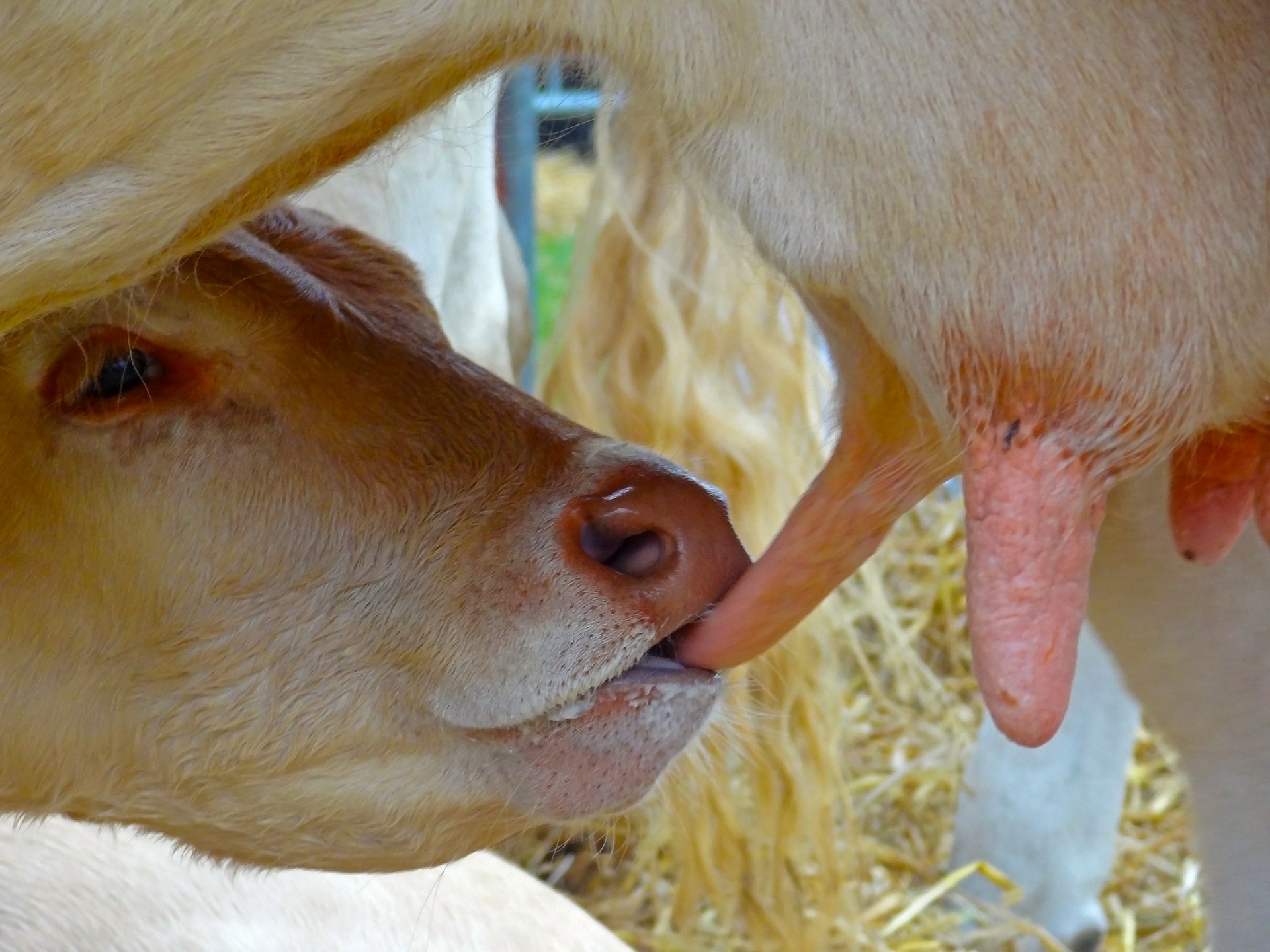
by Technical Team, EW Nutrition
Sub-acute acidosis (SARA) is linked to high levels of ruminal LPS. The LPS cause inflammation and contribute to different metabolic conditions and diseases. Various strategies and solutions can be applied to modulate the rumen microbiota and prevent this risk.
In sub-acute rumen acidosis (SARA), the quantity of free lipopolysaccharides (LPS) coming from Gram- bacteria increases considerably. These LPS cross the ruminal wall and intestine, passing into the bloodstream. The negative consequences on the health of the animal are then reflected in decreased productive and reproductive performance.
The LPS are released during the lysis of GRAM- bacteria which die due to the low pH, and these bacteria are mainly responsible for the production of propionic acid for the energy yield that is obtained. It is essential to preserve ruminal balance between Gram+ and Gram- such that there is no excess of LPS.
Nutritional needs of lactating cows with SARA
In the first phase of lactation (from 1 week after calving to 80 – 100 days of lactation), the cow needs a high energy level to meet the large demand for milk production. This energy demand is often not fully satisfied and feed intake falls short. This deficit leads to the need to provide as much energy as possible per feed ration.
Imagine a 650 kg live weight cow, producing about 35 kg of milk per day with a fat percentage of 3.7 and a protein percentage of 3.2. To achieve this production level and fulfill its maintenance requirements, this animal needs a feed intake of 22 kg of dry matter (DM) per day, with an energy level of 21 UFL equal to 36,000 Kcal/day of NE l (Net Energy Lactation)).
To obtain an energy supply of this type, it is necessary to provide rations with a high content of cereals rich in nonstructured carbohydrates (NSC). This will allow the animals to obtain the maximum efficacy in getting the NE I from the metabolizable energy (ME) expressed as kl*.
*kl expresses the effectiveness in passing from EM to EN l net of the heat dissipated by the animal, therefore kl = ENl/EM (Van Es 1978).
Compared to a diet rich in NDF (Neutral Detergent Fiber), this type of diet promotes and stimulates certain strains of bacteria to the detriment of others, shifting the balance towards a greater population of bacteria that produce propionic acid instead those which produce acetic acid. This change also determines a greater share of Gram- compared to Gram+.
What is rumen acidosis?
Rumen acidosis is that “pathology” whereby the volume of SCFA (Short Chain Fatty Acids) produced by the rumen bacteria is greater than the ability of the rumen itself to absorb and neutralize them. Rumen acidosis is mainly caused by the amylolytic and saccharolytic bacteria (Streptococcus bovis; Selenomonas ruminantium, Bacteroides amylophilus, Bacteroides ruminicola and others) responsible for the production of lactic acid. Unlike the other most representative volatile fatty acids (acetic, butyric and propionic), lactic acid has a lower pKa: 7 (3.9 versus 4.7). This means that for the same amount of molecules produced, lactic acid releases a number of ions H+ in the fluid ten times greater than other VFAs, with evident effects on the pH.
Ruminal acidosis can be characterized as acute or subacute. During acute ruminal acidosis, the pH in the rumen drops below 4.8 and remains low for an extended period of time. Acute acidosis leads to complete anorexia, abdominal pain, diarrhea, lethargy, and eventually death. However, the prevalence of acute acidosis in dairy is very low.
Consequences of rumen acidosis
In such situations, a series of negative consequences can be triggered in the lactating cow. Investigations (for instance, using fistulated cows) can reveal, among others, the following alteration in the rumen:
- Shift in total microbiome rumen profile (density; diversity; community structure)
- Shift in protozoa population (increase in ciliates protozoa after 3 weeks of SARA; increase in the GNB population)
- Shift in fungi population (decreasing the fungi population with high fibrolytic enzymes, which are sensitive to low pH)
- Rise in LPS rumen concentration (increasing the GNB strain and their lysis)
- Influence on the third layer of Stratified Squamous Epithelium (SSE) (desmosomes and tight junctions)
- Lower ruminal fiber degradation (reduction in the number of cellulolytic bacteria which are less resistant to acid pH)
- Reduction of the total production of fatty acids (propionic, acetic, butyric), therefore less available energy
- Lower rumen motility (also as a consequence of the smaller number of protozoa)
- The increased acid load damages the ruminal epithelium
- Acid accumulation increases the osmotic pressure of the rumen inducing an higher flux of water from the blood circulation into the rumen, causing swelling and rupture of rumen papilla as well as a greater hemoconcentration
The last points are extremely important, as it enables an easier passage of fluids from the blood to the pre-stomachs, greatly influencing the fermentation processes.
Furthermore, with diets low in NDF, the level of chewing and salivation is certainly lower, with a consequent lower level of salivary buffers that enter the rumen and which would maintain an appropriate pH under normal conditions.
Rumen sub-acute and acute acidosis: a fertile ground for LPS
Studies inducing SARA in dairy cows have shown that feeding high levels of grain causes the death and cell lysis of Gram- bacteria, resulting in higher concentration of free LPS in the rumen. In a trial conducted by Ametaj et al., in 2010 (Figure 1), a lower ruminal pH and an increase in the concentration of LPS in the rumen fluid -measured as ng / ml (nanograms / milliliter)-, was the result of increasing of NSC present in the diet (% of grains).
In the rumen, the presence of Gram- is very significant, however the dietary changes towards high energy concentrates, reduce the substates necessary for them to thrive, leading to their lysis and favoring gram-positive bacteria (Gram+). Gram+ also produce bacteriocins against a wide variety of bacteria, including many Gram-. Figure 2 shows the influence of ruminal pH in the population of different bacteria, many of which are are crucial for the production of SCFA and therefore of energy.
It is therefore necessary to pay close attention to the energy level of the ration as an energy input (generally around 1500 – 1700 Kcal/kg of DM intake). At the same time, we need to ensure that the animal does receive and ingest that daily amount of DM. If ingestion is negatively influenced by acidosis (clinical or sub-clinical), this can lead to endotoxemia, with harmful consequences for the animal’s health and production performance.
We can therefore note that the level of LPS (endotoxins) present in the rumen is directly correlated with the pH of the rumen itself and with a symptomatologic picture dating back to SARA. This occurs when the mortality and lysis of Gram- bacteria (GNB) is high and through the consequent imbalance created with diets containing excess fermentable starches, compared to diets with higher fiber content.
In fact, it was shown that the transition from a concentrated fodder ratio of 60:40 to a more stringent ratio of 40:60 caused the level of free LPS in the rumen to go from 410 to 4.310 EU / ml.
Endotoxemia: Pathological consequences in dairy cows
Once the LPS enter the bloodstream, they are transported to the liver (or other organs) for the detoxification. However, sometimes this is not enough to neutralize all the endotoxins present in blood. The remaining excess can cause issues such as the modification of the body’s homeostasis or cause that cascade of inflammatory cytokines responsible for the most common pathologies typical in cows in the first phase of lactation. The most common symptoms are the increase of somatic cells in milk or claws inflammation.
Pro-inflammatory cytokines as TNF, IL6 and IL8 induced by LPS-related inflammation are able to stimulate the production of ACTH (adrenocorticotropic hormone).
ACTH, together with cortisol and the interleukins, inhibit the production of GnRH and LH, with serious effects on milk production. The productivity and the fertility of the animal are thus compromised.
Moreover, prostaglandins are as well stimulated by LPS, and are linked with fever, anorexia and ruminal stasis. This not only limits the amount of energy available for production and maintenance functions, but also induces a higher susceptibility to disease and adds-up to the emergence of other metabolic conditions, such as laminitis and mastitis.
Preventing rumen acidosis
The solution to these massive risks is a prudent and proactive approach by the nutritionist towards all situations that can cause a rapid increase of Gram- in the rumen. It is therefore necessary to avoid cases of clinical and sub-clinical acidosis (SARA) in order to avoid the issues listed above. This would also help avoid stressful conditions for the animal that would lead to decreased performance and health.
To maintain balance and a healthy status of the animal, the use of additives such as phytomolecules and binders is suggested in the first phase of lactation, starting from 15 days before giving birth.
Activo Premium (a mix of phytogenic substances) has given excellent results in decreasing the acetic/propionic acid ratio, while safeguarding the population of Gram+ bacteria. This is in contrast to treatments with ionophores, which, as is well known, interfere with the Gram+ population.
Case study. Acetic acid:propionic acid ratio with Activo Premium
In a study conducted at the the University of Lavras and the Agr. Res. Comp. of Minas Gerais (both Brazil), 30 Holstein cows were allocated to two groups considering parity and milk production. One group was fed the standard feed (control), the other group received standard feed containing 150mg of Activo Premium/kg of dietary dry mass (DM). The following parameters were measured or calculated: intake of DM and milk production, milk ingredients such as fat, protein, lactose every week, body weight and body condition score every two weeks, and ruminal constituents (ph and SCFAs) through oesophaeal samples at day 56.
Activo Premium was able to decrease the ratio between acetic acid and propionic acid, and at the same time maintain the level of Gram+ bacteria in the rumen, thus reducing the risk of endotoxins. The same trial carried out at the University of Lavras demonstrated how the performance of the animals was superior in the group fed with Activo Premium compared to the control group (see below).
Solution: Preserve Gram+ bacteria levels while decreasing free LPS
We have therefore seen how important it is to decrease the acetic:propionic ratio in the rumen to obtain a greater share of available energy. However, the level of endotoxins in the rumen must remain low in order to avoid those problems of endotoxemia linked to very specific pathologies typical of “super productive cows”. These pathologies (always linked to inflammatory manifestations) can be prevented by decreasing the level of free LPS in the rumen with a product that can irreversibly bind the LPS and thus make them inactive.
In a trial with porcine intestinal cells (IPEC-J2) challenged by E. coli LPS, a decrease in the intensity of inflammation was observed when Mastersorb Gold was added. This decrease could be shown through a lower amount of phosphorylated NF-kB in an immunofluorescence trial, as well as through the reduced production of Interleukin (IL)-8 in the cells measured by ELISA.
The fact that pig intestine tissue was used does not affect the adsorption concept. In this case, these intestinal cells are only a vehicle to demonstrate that in an aqueous solution containing 50 ŋg of LPS / ml and in the same solution with the addition of Mastersorb Gold, the level of LPS actually active is decreased, as a part of the LPS was tied up by Mastersorb. The solution with a lower level of LPS gave minor “inflammatory” reactions to intestinal cells, and this can be statistically reported in dairy cows.
Conclusions
To demonstrate how the decrease in the level of LPS in the rumen is directly correlated with inflammatory states in general, a trial with a total of 60 dairy cows shows that the inclusion of 25g of Mastersorb Premium/animal/day increases milk yield and improves milk quality by decreasing somatic cell count. Adsorbing substances contained in Mastersorb Premium tie up the LPS produced in the rumen in different cow lactation phases.
Normally, the rise in the level of somatic cells in milk depends on etiological agents such as Streptococcus spp, Staphylococcus spp, mycoplasma and more. LPS stress is not the sole agent responsible for raising somatic cell counts, but also other factors among which:
- Lactation stage and age of the animal
- Season of the year (in summer the problem is increased)
- Milking plant (proper maintenance)
- General management and nutrition
However, by reducing the level of LPS, Mastersorb provides an important aid to decrease somatic cell count.
Prevent escalation with rumen balance
In the end, ruminant producers are, like all livestock operations, interested in producing healthy animals that can easily cope with various stressors. Ensuring a proper diet, adjusted to the energy requirements of various production stages, is a first step. Providing the animal with the ingredients that modulate the microbiota and reduce the negative impact of stress in the rumen is the next essential step in efficient production.