Mycotoxins as contributors to antibiotic resistance?
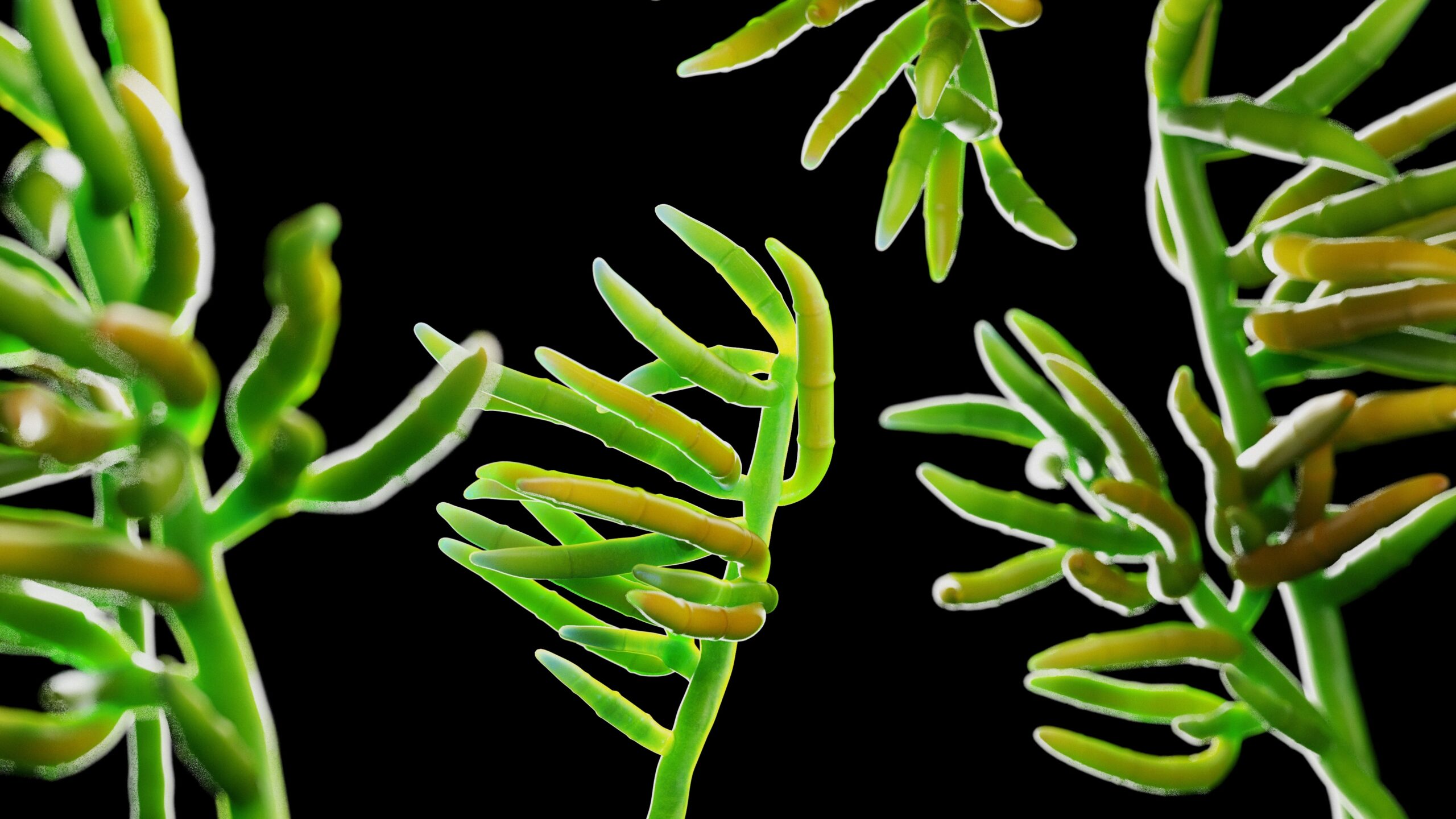
By Dr. Inge Heinzl, Editor EW Nutrition and
Marie Gallissot, Global Manager Feed Quality Solutions EW Nutrition
Antibiotic resistance is a growing global health concern, making infections more complicated to treat and increasing the risk of disease spread, severe illness, and death. While overuse and misuse of antibiotics are the primary causes, recent research has uncovered another unexpected contributor: mycotoxins. Among these, deoxynivalenol (DON), a toxin commonly found in contaminated grains, has been shown to significantly alter gut microbiota and promote antibiotic resistance. This article examines how DON impacts gut bacteria, influences antibiotic resistance, and highlights why this issue warrants urgent attention.
Mycotoxins – originators of antimicrobial resistance?
Actually, it would be logical…
Alexander Fleming discovered Penicillin when he returned after the summer holidays and saw that a mold had grown on the agar plate he had prepared. Around the mold, Staphylococcus was unable to proliferate. The reason was a substance produced by the mold – penicillin, which, like other toxins produced by molds, is a mycotoxin. In his article about the origin of antibiotics and mycotoxins, Shier (2011)
stated that antibiotics and mycotoxins share considerable similarities in structure, metabolic roles, and biosynthesis.
A short excursus to antimicrobial resistance
In general, the primary mechanisms of resistance involve the prevention or limitation of the antimicrobial substance’s uptake, modifying the drug target, inactivating the drug, or facilitating its discharge with efflux pumps.
There are two types of resistance: natural resistance, which is further divided into intrinsic and induced resistance, and acquired resistance.
Intrinsic resistance is a “characteristic” of a bacterial species and is not dependent on antibiotic exposure. An example is the reduced permeability of the outer membrane of gram-negative bacteria, which prevents certain antibiotics from entering.
Induced resistance, however, needs to be initiated by antibiotics. Here, multidrug-efflux pumps can be mentioned.
The third one, acquired resistance, refers to the process by which bacteria acquire genetic material, the resistance genes, from other bacteria that are resistant. The mechanisms include vertical transfer to daughter cells and horizontal transfer, such as the transfer from dead bacteria to living ones, by viruses, or the transfer of plasmids (Reygaert, 2018).
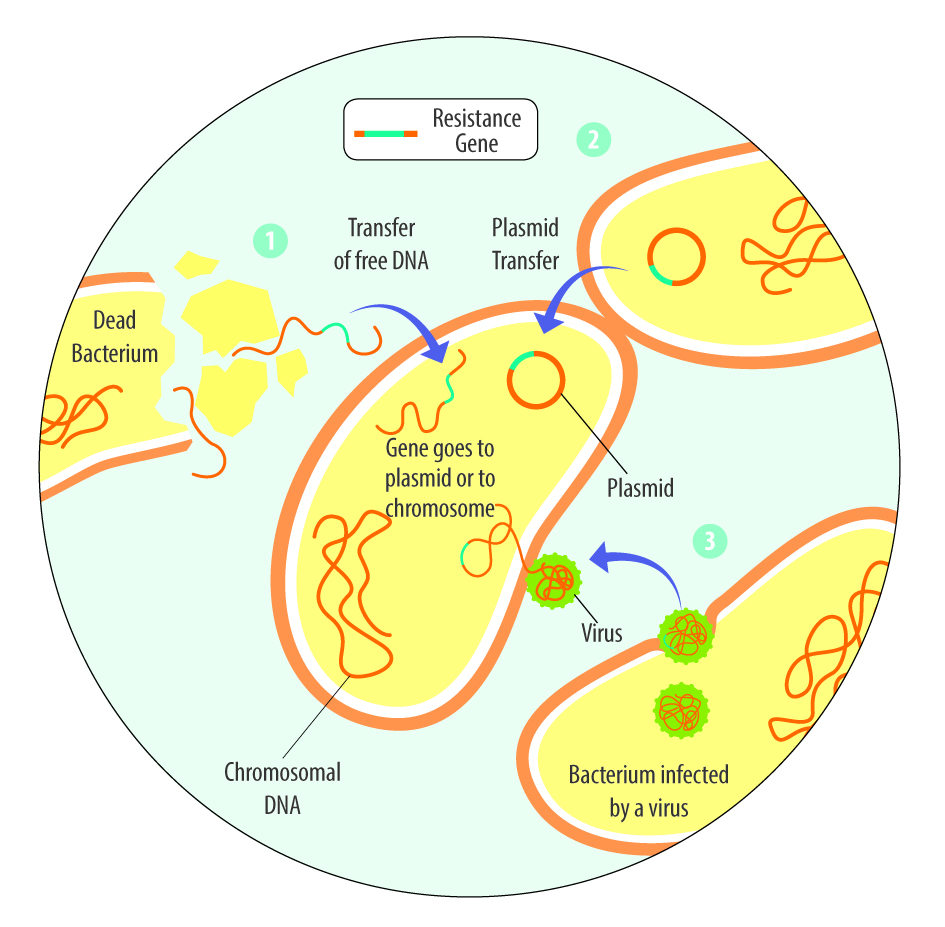
Deoxynivalenol (DON) promotes resistance in gut microbiota
A Chinese group of researchers (Deng et al., 2025) examined for the first time the influence of DON on the intestinal microbiota of chickens. One of the most alarming findings is DON’s ability to enhance antibiotic resistance. It contributes to this issue in several ways:
- Encouraging resistant bacteria – By disrupting microbial balance, DON provides a survival advantage to bacteria that carry resistance genes.
- Activating resistance genes – Studies suggest that DON can increase the expression of genes that help bacteria withstand antibiotics.
- Enhancing gene transfer – Bacteria can share resistance genes through horizontal gene transfer. DON appears to promote this process, making antibiotic-resistant strains spread more rapidly.
- Weakening antibiotic effectiveness – DON-induced changes in the gut environment can reduce the effectiveness of antibiotics, making treatments less successful.
A further indication that mycotoxins can enhance resistance is the significant overlap in the geographical distribution of antimicrobial-resistant bacteria and genes with that of mycotoxins, as noted by Deng et al.
Which protection mechanisms do bacteria have against mycotoxins?
In the case of mycotoxins, bacteria employ similar molecular mechanisms to those used against antibiotics. In an in vitro experiment, Hassan et al. (2019) challenged Devosia mutans, a gram-negative bacterium, with DON in the growth medium. DON inhibits protein synthesis, induces oxidative stress, and compromises cell membrane integrity in eucaryotic cells. Hassan et al. asserted three adaptive mechanisms as the response to the challenge:
- Activation of cellular membrane proteins (adenosine 5’-triphosphate-binding cassette -ABC- transporters) responsible for the unidirectional transport of substrates, either outward or inward. These ABC transporters can work as drug efflux pumps.
- Production of DON-specific deactivation enzymes, thereby engaging a toxin-specific pyrroloquinoline quinone-dependent detoxification pathway. This enables the bacterial isolate to transform DON to a non-toxic stereoisomer.
- Upregulation of auxiliary coping proteins, such as porins (transmembrane proteins involved in metabolite exchange), glutathione S-transferases, and phosphotransferases, both of which are likely involved in the detoxification of xenobiotics.
Public health implications and preventive measures
Given the widespread presence of DON in food and animal feed, its potential role in antibiotic resistance poses a serious threat. The combination of increased bacterial resistance and weakened antibiotic efficacy could lead to more difficult-to-treat infections. This is particularly concerning in hospital settings, where antibiotic-resistant infections already cause high mortality rates.
To address the issue, several strategies can be implemented:
- Reducing DON contamination: Implementing improved agricultural practices, such as crop rotation, the use of fungal-resistant crop varieties, and maintaining proper storage conditions, can help limit fungal growth and DON production.
- Monitoring food and feed supply – Strict regulations and testing for DON contamination in grains and animal feed are essential to minimize human and animal exposure.
- Effective mycotoxin risk management at feed mill and farm levels: Using tools such as MasterRisk and effective products combatting mycotoxins.
- Maintaining gut health: A healthy diet rich in fiber, probiotics, and gut health-supporting feed supplements, such as Ventar D or products from the Activo line, may help counteract some of the adverse effects of DON on gut microbiota.
- Developing new treatments: Research into alternative therapies and new antibiotics is crucial to combat the rise of antibiotic resistance.
Antimicrobial resistance: Be aware of the mycotoxins!
The connection between mycotoxins, such as DON, and antibiotic resistance underscores the need for a broader perspective on public health and food safety and once again brings the “One Health Concept” into focus. While antibiotic overuse remains the primary driver of resistance, environmental factors, such as exposure to mycotoxins, should not be overlooked. By increasing awareness, enhancing food safety regulations, and investing in research, we can take steps to mitigate this emerging threat and safeguard the effectiveness of antibiotics for future generations.
References:
Deng, Fengru, Chuying Yao, Linyu Ke, Meichan Chen, Mi Huang, Jikai Wen, Qingmei Chen, Jun Jiang, and Yiqun Deng. “Emerging Threat to Antibiotic Resistance: Impact of Mycotoxin Deoxynivalenol on Gut Microbiota and Clonal Expansion of Extensively Drug-Resistant Enterococci.” Environment International 197 (March 2025): 109353.
https://doi.org/10.1016/j.envint.2025.109353.
Hassan, Yousef I., Jian Wei He, Dion Lepp, and Ting Zhou. “Understanding the Bacterial Response to Mycotoxins: The Transcriptomic Analysis of Deoxynivalenol-Induced Changes in Devosia Mutans 17-2-E-8.” Frontiers in Pharmacology 10 (November 14, 2019).
https://doi.org/10.3389/fphar.2019.01098.
Reygaert, Wanda C. “An Overview of the Antimicrobial Resistance Mechanisms of Bacteria.” AIMS Microbiology 4, no. 3 (2018): 482–501.
https://doi.org/10.3934/microbiol.2018.3.482.
Shier, W. Thomas. “On the Origin of Antibiotics and Mycotoxins.” Toxin Reviews 30, no. 1 (January 28, 2011): 6–30.
https://doi.org/10.3109/15569543.2011.550862.
Smith, William P., Benjamin R. Wucher, Carey D. Nadell, and Kevin R. Foster. “Bacterial Defences: Mechanisms, Evolution and Antimicrobial Resistance.” Nature Reviews Microbiology 21, no. 8 (April 24, 2023): 519–34.
https://doi.org/10.1038/s41579-023-00877-3.