The crucial role of short-chain fatty acids and how phytomolecules influence them
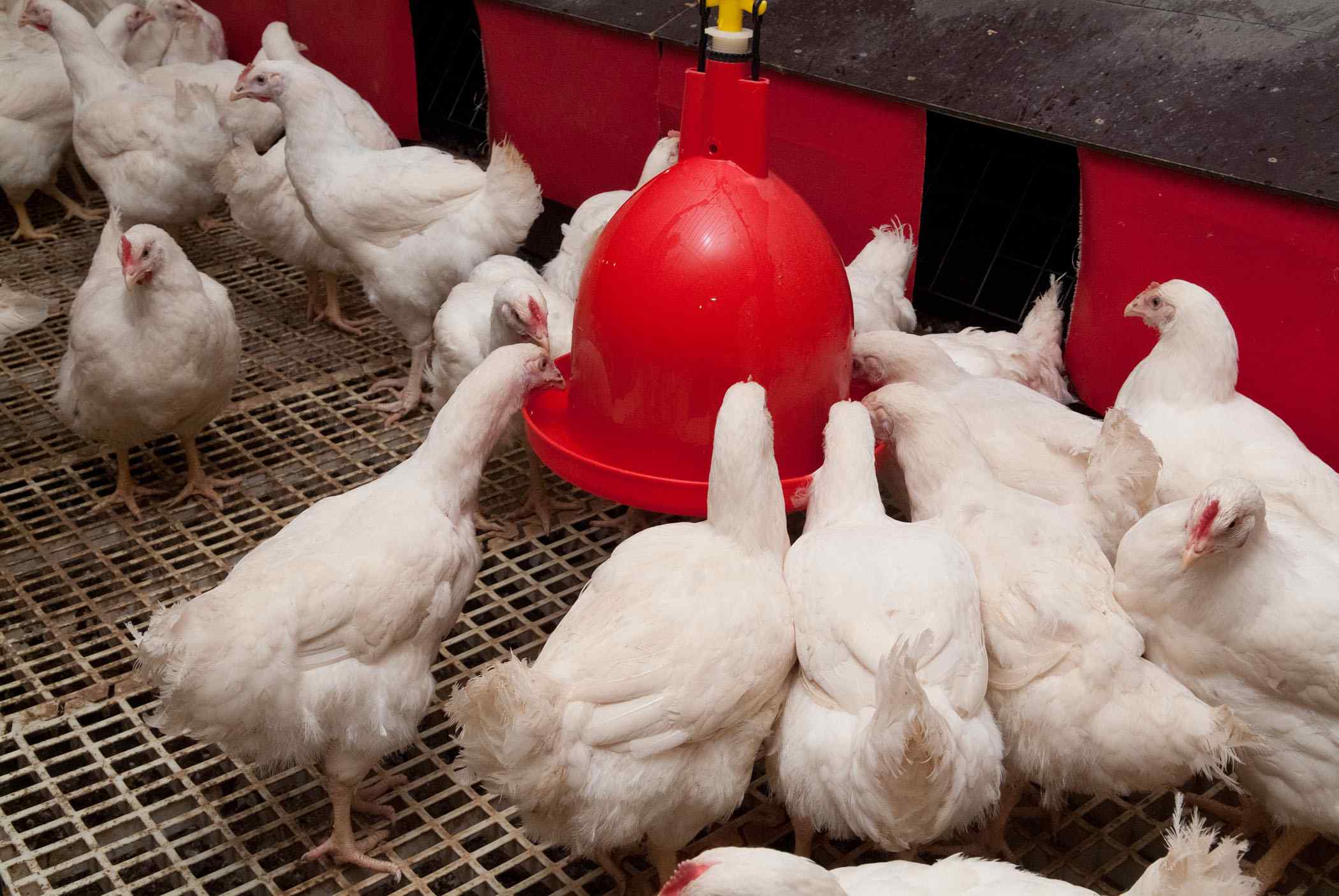
by Dr. Inge Heinzl, Editor EW Nutrition
For optimum health, the content of short-chain fatty acids (SCFAs) is decisive. On the one hand, they act locally in the gut, on the other hand, they are absorbed via the intestinal mucosa into the organism and can affect the whole body. Newer studies in humans show a connection between the deficiency of SCFAs and the occurrence of chronic diseases such as diabetes type 2 or chronic inflammatory gut diseases.
SCFAs – what are they, and where do they come from?
SCFAs consist of a chain of one to six carbon atoms. They are crucial metabolites primarily generated through the bacterial fermentation of dietary fiber (DF) in the hindgut. However, SCFAs and branched SCFAs can also arise during protein fermentation. Short-chain fatty acids predominantly include acetate, propionate, and butyrate, which together account for over 95% of the total SCFAs, typically in a 60:20:20 ratio.
Acetate is produced in two different ways, via the acetyl-CoA and the Wood-Ljungdahl pathways where Bacteroides spp., Bifidobacterium spp., Ruminococcus spp., Blautia hydrogenotrophica, Clostridium spp. are involved. Additionally, acetogenic bacteria can synthesize acetate from carbon dioxide and formate through the Wood-Ljungdahl pathway (Ragsdale and Pierce, 2021). Acetate counts for more than 50% of the total SCFAs in the colon and is the most abundant one.
Propionate can also be produced in two ways. If it is produced via the succinate pathway involving the decarboxylation of methyl malonyl-CoA, the essential bacteria are Firmicutes and Bacteroides. In the acrylate pathway, lactate is converted to propionate. Here, only some bacteria, such as Veillonellaceae or Lachnospiraceae, participate.
Butyrate is produced from acetyl-CoA via the classical pathway by several Firmicutes. However, also other gut microbiota such as Actinobacteria, Proteobacteria, and Thermotogae, which contain essential enzymes (e.g., butyryl coenzyme A dehydrogenase, butyryl-CoA transferase, and butyrate kinase) can be involved. Butyrate can also be produced via the lysine pathway from proteins.
Besides the production of SCFAs from dietary fiber, there is another possibility for the synthesis of SCFAs as well as branched SCFAs – the fermentation of protein in the hindgut. This is something we want to avoid, since it´s clear signal of incorrect animal nutrition. It tells us that there is either oversupply of protein or decrease in protein digestion and absorption.
Which roles do SCFAs play?
SCFAs play a crucial role in the maintenance of gut health. Some benefits originate from these substances’ general character, while others are specific to one acid. If we talk about the benefits of all SCFAs, we can mention the following:
- Primarily, SCFAs are absorbed by the intestine and serve enterocytes as an essential substrate for energy production.
- By lowering the pH in the intestine, SCFAs inhibit the invasion and colonization of pathogens.
- SCFAs can cross bacterial membranes in their undissociated form. Inside the bacterial cell, they dissociate, resulting in a higher anion concentration and bactericidal effect (Van der Wielen et al., 2000)
- SCFAs repair the intestinal mucosa
- They mitigate intestinal inflammation by G protein-coupled receptors (GPRs).
- They enhance immune response by producing cytokines such as IL-2, IL-6, IL-10, and TNF-α in the immune cells. Furthermore, they enhance the differentiation of T-cells into T regulatory cells (Tregs) and bind to receptors (Toll-like receptor, G protein-coupled receptors) on immune cells (Liu et al., 2021).
- SCFAs are involved in the modulation of some processes in the gastrointestinal tract, such as electrolyte and water absorption (Vinolo et al., 2011)
After seeing the general characteristics of short-chain fatty acids, let us take a closer look at the specialties of the single SCFAs.
Acetate might play a crucial role in the competitive process between enteropathogens and bifidobacteria and help to build a balanced gut microbial environment (Liu et al., 2021). Additionally, acetate promotes lipogenesis in adipocytes (Liu et al., 2022).
Concerning general health, acetate inhibits, e.g., lung inflammatory response and the reduced air-blood permeability induced by avian pathogenic E. coli-caused chicken colibacillosis (Peng et al., 2021).
Propionate is thought to be involved in controlling intestinal inflammation by regulating the immune cells assisting and, consequently, in maintaining the gut barrier. Furthermore, propionate regulates appetite, controls blood glucose, and inhibits fat deposition in broiler chickens (Li et al., 2021).
In a trial conducted by Elsherif et al. (2022), birds fed a diet with 1.5 g sodium propionate/kg showed considerably (P<0.05) longer and wider guts, higher counts of lactobacillus(P<0.05) and no colonization of Clostridium perfringens. The immunological state improved significantly (P<0.05), which could be seen by the higher antibody titers when the birds were vaccinated against Newcastle disease or avian influenza.
Butyrate additionally improves the function of the intestinal barrier by regulating the assembly of tight junctions (Peng et al., 2009) and stimulating cell renewal and differentiation of the enterocytes. Butyrate-producing microbes on their side prevent the dysbiotic expansion of potentially pathogenic E. coli and Salmonella (Byndloss et al., 2017; Cevallos et al., 2021) by stimulating PPAR-γ signaling. This leads to the suppression of iNOS synthesis and a significant reduction of iNOS and nitrate in the colonic lumen. Furthermore, the microbiota-induced PPAR-γ-signaling inhibits dysbiotic Enterobacteriaceae expansion by limiting the bioavailability of oxygen and, therefore, respiratory electron acceptors to Enterobacteriaceae in the colon.
In a trial conducted by Xiao et al. (2023), sodium butyrate enhanced broiler breeders’ reproductive performance and egg quality due to the regulation of the maternal intestinal barrier and gut microbiota. Additionally, it improved the antioxidant capacity and immune function of the breeder hens and their offspring.
SCFAs’ production can be managed
The extent of production depends on the diet and the composition of the intestinal flora. Nutritional strategies can be taken to regulate the production of short-chain fatty acids by providing dietary fiber and prebiotics, the respective bacteria but also additives in the diet or, on the other, negative way, use of antibiotics.
One example of SCFA-promoting additives is phytomolecules. Ventar D, a blend of diverse gut health-promoting phytomolecules, shows its SCFAs-increasing effect in a trial with Ross 308 broilers.
Trial design: The 41-day research study was conducted at an R&D farm in Turkey, with 3200 Ross 308 broilers in total. The day-old broiler chicks were randomly divided into two groups with 8 replicates in 16-floor pens (6.5×2 m each), each of 200 chicks (100 males and 100 females). One group was managed as a control group with regular feed formulation, and the other group was supplemented with Ventar D. All the birds were provided feeds and water ad libitum. Temperature, lighting, and ventilation were managed as per Ross 308 recommendation.
Groups | Application dose | |
Starter (crumbles) | Grower & Finisher – 1 & 2 (pellet) | |
Control | No additive | |
Ventar D | 100 gm/MT | 100 gm/MT |
All the birds and feed were weighed on days 0, 11, 23, and 41. Dead birds were also weighed, and the feed consumption was corrected accordingly. At the end of the experiment, one male and one female chicken close to the average weight of each pen were separated, weighed, and slaughtered. Short-chain fatty acid (SCFA) concentration in the caecum was measured by gas chromatography (Zhang et al. 2003). Statistical analysis of the data obtained in this study was carried out in the Minitab 18 program using the T-test following the randomized block trial design (P ≤ 0.05). The research results were subjected to statistical analysis on a pen basis. Mortality results were evaluated with the Chi-square test.
Results: Ventar D significantly increased the levels of acetate, butyrate, and total SCFAs. The level of propionate was numerically higher. Additionally, higher final body weights (on average 160 g), improved feed efficiency (6 points), a higher EPEF (33 points), and lower mortality (0.5%) could be asserted in this experiment.
One explanation could be the microbiota-balancing effect of Ventar D. Meimandipour et al. (2010), for example, saw in their study that increased colonization of Lactobacillus salivarius and Lactobacillus agilis in cecum significantly increased propionate and butyrate formation in caeca.
Phytomolecules: Balancing intestinal microbiome and increasing healthy SCFAs
By promoting beneficial intestinal bacteria and fighting the harmful ones, phytomolecules drive the microbiome in the right direction and promote the production of short-chain fatty acids. Their gut health-protecting effect, in turn, provides for adequate digestion and absorption of nutrients, leading to optimal feed conversion and growth rates. The support of the immune system and the promotion of the antioxidant capacity additionally enhance the health of the animals. Healthy animals grow better, which ultimately leads to a higher profit for the farm.
References:
Byndloss, Mariana X., Erin E. Olsan, Fabian Rivera-Chávez, Connor R. Tiffany, Stephanie A. Cevallos, Kristen L. Lokken, Teresa P. Torres, et al. “Microbiota-Activated PPAR-γ Signaling Inhibits Dysbiotic Enterobacteriaceae Expansion.” Science 357, no. 6351 (August 11, 2017): 570–75. https://doi.org/10.1126/science.aam9949.
Cevallos, Stephanie A., Jee-Yon Lee, Eric M. Velazquez, Nora J. Foegeding, Catherine D. Shelton, Connor R. Tiffany, Beau H. Parry, et al. “5-Aminosalicylic Acid Ameliorates Colitis and Checks Dysbiotic Escherichia Coli Expansion by Activating PPAR-γ Signaling in the Intestinal Epithelium.” mBio 12, no. 1 (February 23, 2021). https://doi.org/10.1128/mbio.03227-20.
Elsherif, Hany M.R., Ahmed Orabi, Hussein M.A. Hassan, and Ahmed Samy. “Sodium Formate, Acetate, and Propionate as Effective Feed Additives in Broiler Diets to Enhance Productive Performance, Blood Biochemical, Immunological Status, and Gut Integrity.” Advances in Animal and Veterinary Sciences 10, no. 6 (June 2022): 1414–22.
Li, Haifang, Liqin Zhao, Shuang Liu, Zhihao Zhang, Xiaojuan Wang, and Hai Lin. “Propionate Inhibits Fat Deposition via Affecting Feed Intake and Modulating Gut Microbiota in Broilers.” Poultry Science 100, no. 1 (January 2021): 235–45. https://doi.org/10.1016/j.psj.2020.10.009.
Liu, Lixuan, Qingqing Li, Yajin Yang, and Aiwei Guo. “Biological Function of Short-Chain Fatty Acids and Its Regulation on Intestinal Health of Poultry.” Frontiers in Veterinary Science 8 (October 18, 2021). https://doi.org/10.3389/fvets.2021.736739.
Liu, Lixuan, Qingqing Li, Yajin Yang, and Aiwei Guo. “Biological Function of Short-Chain Fatty Acids and Its Regulation on Intestinal Health of Poultry.” Frontiers in Veterinary Science 8 (October 18, 2021). https://doi.org/10.3389/fvets.2021.736739.
Meimandipour, A., M. Shuhaimi, A.F. Soleimani, K. Azhar, M. Hair-Bejo, B.M. Kabeir, A. Javanmard, O. Muhammad Anas, and A.M. Yazid. “Selected Microbial Groups and Short-Chain Fatty Acids Profile in a Simulated Chicken Cecum Supplemented with Two Strains of Lactobacillus.” Poultry Science 89, no. 3 (March 2010): 470–76. https://doi.org/10.3382/ps.2009-00495.
Peng, Lu-Yuan, Hai-Tao Shi, Zi-Xuan Gong, Peng-Fei Yi, Bo Tang, Hai-Qing Shen, and Ben-Dong Fu. “Protective Effects of Gut Microbiota and Gut Microbiota-Derived Acetate on Chicken Colibacillosis Induced by Avian Pathogenic Escherichia Coli.” Veterinary Microbiology 261 (October 2021): 109187. https://doi.org/10.1016/j.vetmic.2021.109187.
Peng, Luying, Zhong-Rong Li, Robert S. Green, Ian R. Holzmanr, and Jing Lin. “Butyrate Enhances the Intestinal Barrier by Facilitating Tight Junction Assembly via Activation of AMP-Activated Protein Kinase in Caco-2 Cell Monolayers.” The Journal of Nutrition 139, no. 9 (September 2009): 1619–25. https://doi.org/10.3945/jn.109.104638.
Ragsdale, Stephen W., and Elizabeth Pierce. “Acetogenesis and the Wood–Ljungdahl Pathway of CO2 Fixation.” Biochimica et Biophysica Acta (BBA) – Proteins and Proteomics 1784, no. 12 (December 2008): 1873–98. https://doi.org/10.1016/j.bbapap.2008.08.012.
Vinolo, Marco A.R., Hosana G. Rodrigues, Renato T. Nachbar, and Rui Curi. “Regulation of Inflammation by Short Chain Fatty Acids.” Nutrients 3, no. 10 (October 14, 2011): 858–76. https://doi.org/10.3390/nu3100858.
Wielen, Paul W. van der, Steef Biesterveld, Servé Notermans, Harm Hofstra, Bert A. Urlings, and Frans van Knapen. “Role of Volatile Fatty Acids in Development of the Cecal Microflora in Broiler Chickens during Growth.” Applied and Environmental Microbiology 66, no. 6 (June 2000): 2536–40. https://doi.org/10.1128/aem.66.6.2536-2540.2000.
Xiao, Chuanpi, Li Zhang, Bo Zhang, Linglian Kong, Xue Pan, Tim Goossens, and Zhigang Song. “Dietary Sodium Butyrate Improves Female Broiler Breeder Performance and Offspring Immune Function by Enhancing Maternal Intestinal Barrier and Microbiota.” Poultry Science 102, no. 6 (June 2023): 102658. https://doi.org/10.1016/j.psj.2023.102658.